Latest development of nanostructured si/c materials for lithium anode studies and applications
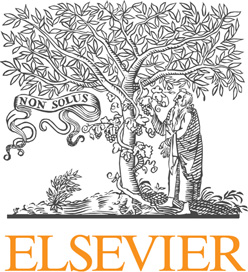
Contents lists available at
Energy Storage Materials
journal homepage:
Latest development of nanostructured Si/C materials for lithium anodestudies and applications
Miao Zhang Tengfei Zhang , Yanfeng Ma Yongsheng Chen
a Centre for Nanoscale Science and Technology, Collaborative Innovation Center of Chemical Science and Engineering (Tianjin), School of Materials Scienceand Engineering, Nankai University, Tianjin 300071, Chinab Key Laboratory of Functional Polymer Materials and the Institute of Polymer Chemistry, College of Chemistry, Nankai University, Tianjin 300071, China
Silicon anodes for lithium-ion batteries have been extensively explored due to their high capacity, moderate
Received 15 November 2015
operation potential, environmental friendliness, and high abundance. However, silicon's application as
Received in revised form
anodes is hindered by its poor capacity retention caused by the large volume change during lithium insertion
and desertion process, its intrinsic low conductivity and the formation of unstable solid-electrolyte inter-
Accepted 1 February 2016
phase (SEI) films. Recently, influential improvements have been achieved using different design methods
Available online 12 February 2016
with the purpose of increasing cycle life and increasing charging rate performance. Here, we review such
design methods including the rational design of nanostructured silicon, the combination of silicon with
Lithium ion batteries
different carbonaceous materials including traditional carbons and the utilization of nanocarbons (such as
carbon nanotube, graphene and corresponding three dimensional architectures). Meanwhile, we draw the
essential reason accounting for the excellent electrochemical performance of those structures. Furthermore,
Carbon nanotubesGraphene
we selectively depict the effects of binder, conductive additives and electrolyte composition, which also playimportant roles in silicon based battery performance.
& 2016 Elsevier B.V. All rights reserved.
n Corresponding author at: Centre for Nanoscale Science and Technology, Colla-
borative Innovation Center of Chemical Science and Engineering (Tianjin), School of
Energy is one of the most important topics of the 21st century.
Materials Science and Engineering, Nankai University, Tianjin 300071, China.
Ever rising demands for energy coupled with the depletion of
Fax: þ86 2223 499992.
E-mail address: (Y. Chen).
finite fossil fuel and the emission of contaminative gases have
2405-8297/& 2016 Elsevier B.V. All rights reserved.
M. Zhang et al. / Energy Storage Materials 4 (2016) 1–14
encouraged scientists to develop new energy storage and conver-
pulverization of the Si particles can cause lithium trapping in the
sion technologies for renewable and clean energy sources. Among
active material and progressively consume of active material
various energy storage technologies, electrochemical storage is
during cycling, all of which contribute to low Coulombic efficiency
considered as one of the most promising technologies, especially
and high irreversible capacity. (2) Disruption of the electron–ion
for the applications of electric vehicles (EVs), plug-in hybrid
transport pathways at the level of the entire electrode: The elec-
electric vehicles (PHEVs) and hybrid electric vehicles (HEVs)
trode is composed with Si particles as active materials, carbon
Moreover, the popularization of portable electronics and com-
black to enhance the conductivity and binder to facilitate the
munication equipment worldwide stimulates the development of
integrity of the whole electrode. During lithiation process, the Si
energy storage devices, such as batteries and supercapacitors with
particles expand, suppressing the surrounding materials expand-
higher energy density and higher power density respectively
ing at the same time, which exceed the mechanical elongation of
Lithium ion batteries (LIBs) are widely used as a convenient power
them, resulting in electrical detachment of the surrounding com-
source for various portable electronic devices and are considered
ponents with active materials. Finally, this drastic electrode mor-
as potential energy source to power EVs, HEVs and PHEVs
phology change combined with intrinsic low conductivity disrupt
A LIB is mainly composed of an anode (negative), a cathode
the electron-transport pathways and further contribute to capacity
(positive), a separator, and a certain amount of electrolyte ,
fade. (3) Unstable solid-electrolyte interphase (SEI): When the
The positive electrode materials are typically Li-containing metal
potential of the anode is below ca. 1 V versus Li/Li þ, the decom-
oxides and the negative electrode materials include insertion-type
position of the organic electrolyte at the electrode surface is
materials (such as graphite, Li4Ti5O12 (LTO), etc.), and alloying-type
favorable, forming the SEI layer. If the SEI layer is dense and stable,
materials. The function of the separator is to prevent short cir-
it can prevent further side chemical reactions. But the SEI layer on
cuiting between the cathode and anode electrodes and to provide
the Si surface continuously breaks and the fresh Si unceasingly
abundant channels for transportation of Li ion during charging/
exposes to electrolyte during lithium insertion and desertion,
discharging. The electrolyte should be a good ionic conductor and
leading to extremely thick SEI layers and excessive consumption of
electronic insulator and most of them are based on the solution of
lithium ions and electrolyte. As a consequence of this process,
inorganic lithium salts dissolved in a mixture of organic solvents.
even if most of the Si active materials remain electrically con-
Currently, most commercially available LIBs are made with gra-
nected, the capacity decays because of the poor electronic con-
phite as the anode material and lithium metal oxides/lithium iron
ductivity and the exhaustion of the electrolyte.
phosphate as the cathode material. The theoretical capacities of
To overcome the drawbacks of Si as anode materials, significant
these anode and cathode materials are 372 mAh g1 and ca.
number of achievements have successfully addressed the pro-
200 mAh g1, respectively, resulting in energy density of ca.
blems. There have been several special reviews on Si as anodes for
200 Wh Kg1. However, this energy density value cannot satisfy
LIBs in the recent two years (2014 and 2015) Xu et al.
the demands for higher energy density batteries used in emerging
described the progress in Si-based materials utilized in LIBs in
new-type electronic devices, advanced communication facilities,
terms of composite systems, nanostructure designs, material
and in particular, EVs or HEVs.
synthesis methods, and electrochemical performances . Les-
The energy density of a battery is mainly determined by its
triez et al. summarized the influence of the different parameters of
output voltage and specific capacity, which are dependent on the
the formulation of silicon-based composite electrode on its
electrochemical properties of electrode materials . Alloy type
cyclability . Metal-assisted chemical etching of silicon and
anode materials with high theoretical capacity and low operation
nanoscale silicon materials used as Li-ion battery anodes were
voltage, such as silicon and tin, have been intensively explored to
reviewed by McSweeney1 s group Terranova, Su and Zhu
further increase the energy densities of LIBs for the above-
et al. reviewed Si/C composites respectively ,, but they
mentioned applications . Silicon (Si) is one of the most pro-
did not give specific depiction of the recently reported composites
mising alloy type high capacity alternatives to graphite anodes. Si
of Si with nanocarbons, especially the three dimensional nano-
offers a high theoretical capacity of 4200 mAh g1 because each
carbons. Also, some reviews of progress of LIB materials have
silicon atom can accommodate 4.4 lithium atoms corresponding to
mentioned the progress in Si/C composites . However,
the formation of Li22Si5 alloy. This theoretical specific capacity of
the combination of nanostructured Si and nanostructured carbon
silicon anode is ca. 10 times higher than that of graphite, and ca. 20
in recent years have not been systematically presented. Thus, in
times higher than that of Li4Ti5O12 (LTO). The second merit of Sianodes is its moderate operation potential versus lithium (ca.
this review, we will first summarize the recent progress and
370 mV above Li/Li þ ) . Thirdly, Si is the second most abundant
advances in designing nanostructured Si anode electrode materi-
element on earth and it is environmentally benign. Furthermore,
als. And then, more details for the advantages of combination of
there are large and mature infrastructures for its processing and
above mentioned nanostructured Si with various carbons, espe-
there are growing approaches using cheap raw materials to fab-
cially the nanocarbons, will be presented. Finally, we will move to
ricate nano-Si particles For example, Cui and coworkers
other additional aspects, such as the binder, electrolyte and elec-
recovered Si nanoparticles directly from rice husks, an agricultural
trode additives, which could also impact electrode performance.
waste, in which the silicon naturally exists in the form of silicananoparticles, which show good electrochemical properties whentested as anodes in cells
2. Rational designs of nanoscale dimensional silicon
However, the use of bulk Si experiences large volume changes
(undergoes up to a 300% volume expansion and contraction) as Li
Nanostructured materials improve cycling stability by incor-
ions enter and leave the Si lattice, which shortens the cycle life and
porating pores or voids to accommodate expansion along with
contributes to cell failure The mechanism that affects the
short lithium diffusion distances within the electrode A
electrode lifespan need to be discussed first: (1) Particle pulver-
number of nanoscale morphologies have been investigated to
ization: during the process that lithium ion insert into the Si lat-
minimize electrode pulverization and capacity loss in silicon
tice, the Si particle grow larger and larger and they will collide into
anodes, including zero dimensional (0D) nanoparticles, one
and squeeze each other until attaining three folds of their original
dimensional (1D) nanowires and nanotubes, two dimensional (2D)
volume, leading to extremely large stresses, which cause crac-
thin films and three dimensional (3D) porous structures. In this
king and pulverization of active particles. The cracking and
section, we will discuss them one by one.
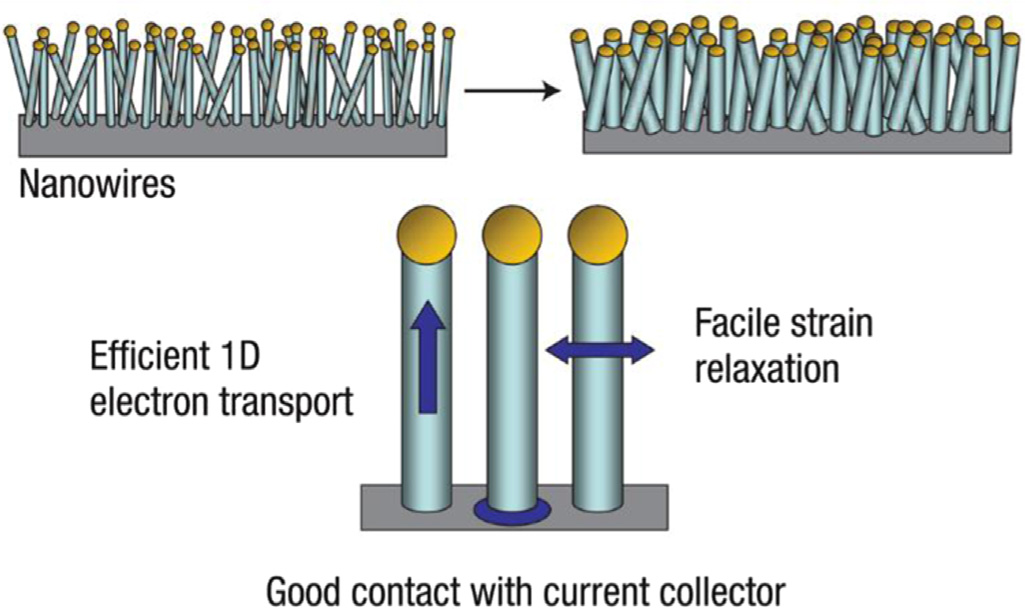
M. Zhang et al. / Energy Storage Materials 4 (2016) 1–14
Nanoscale dimension particles allow quick relaxation of stress,
cycling, leading to superior capacity retention of 89% after 200
making nanoparticles more resistant to fracture than bulk parti-
cycles at a rate of 1 C in practical Li-ion cells . Arrays of sealed,
cles. Silicon with smaller diameters as anodes could cycle more
tubular Si nanotubes, which combine the merits of nanowires and
reversibly with moderately higher capacity than micron-sized
hollow spheres, were expected to further accommodate the large
silicon powder anodes . Some studies have been carried out
volume changes, exhibit high initial Coulombic efficiencies (i.e.,
for the relationship between Si size and the cycle life of Si anode
485%) and stable capacity retention (480% after 50 cycles), due
materials. Kim and coworkers reported 10 nm sized n-Si as the
to an unusual, underlying mechanism that is dominated by free
optimized sample because smaller Si particle encounter with lar-
surfaces An active silicon nanotube surrounded by an ion-
ger unstable SEI and larger Si particles suffer from larger strain
permeable silicon oxide shell can cycle over 6000 times in half
during volume changes . Wang s group reported the critical
cells while retaining more than 85% of their initial capacity,
15 nm Si building block size with SiO as starting materials . As
resulting from a stable SEI . However, such techniques do not
for the preparation methods, Si nanoparticles (Si NPs) could be
fit with standard preparation methods for manufacturing Li-ion
synthesized from reduction of silica or SiCl
battery electrodes because of its high cost, low syntheses effi-
4 disproportion of
silicon monoxide , ball milling from bulk Si . Compared to
ciency. A simple 1D free-standing carbon-coated Si nanofiber
solid structures, hollow structures provide empty interior space
binderless electrode, which was prepared via magnesiothermic
for the volume expansion, which offers lower diffusion-induced
reduction of electrospun SiO2 nanofiber paper produced by an acid
stresses. The finite element modeling results show that the max-
catalyzed polymerization of tetraethyl orthosilicate (TEOS) in-
imum tensile stress in a hollow Si sphere is five times lower than
flight, was reported with a capacity of 802 mAh g1 after 659
that in a solid sphere with an equal volume of Si . The lower
cycles with a Coulombic efficiency of 99.9% 1D Si nanowires
stress values mean that the hollow nanostructures will fracture
are also promising in application of lithium ion batteries.
less readily. The 0D nanoparticles are most promising nanoscale
Two dimensional thin silicon films have demonstrated high
design for the application of Si/C materials, which we will review
capacities and long cycle lives of Si nanostructures The thin
in the following section. The reasons are as follows: (1) 0D
film expands during lithiation along perpendicular direction, while
nanoparticles are easier to combine with carbon materials,
contraction during delithiation occurs both perpendicular to and
including traditional carbons and nanocarbons in simple methods;
in plane with the substrate, leading to cracking of the film after the
(2) 0D nanoparticles are easier to fabricate with low cost in easy
first discharge cycle. After the initial fracture, the active material
can be cycled without additional film cracking . Thickness,
Other than 0D nanoparticles, 1D silicon nanowires (NWs) have
surface morphology, and the interfacial bonding degree between
shown high discharge capacities and stable cycling over tens of
the Si and current collector all have impact on the performance of
cycles with high reversible capacities. An advanced vertical growth
Si thin films as electrode The reversible capacity and
of NW structure design prepared via vapor–liquid–solid method
cycling life decrease with increasing films thickness, whereascapacity and cycle life increase with increasing films roughness.
not only take advantage of small NW diameter to better accom-
Amorphous n-type silicon films with a thickness of 50 nm vacuum
modation of the large volume changes but also electrically connect
deposited onto nickel substrates exhibited an initial capacity of
each Si NW to the current collector to prompt efficient charge
approximately 3750 mAh g1 and without significant capacity
transport (). When those nanowires were charged with C/5
decay after 200 cycles at 1 C Thicker film of 275 nm delivered
rate, the capacity was stable at ca. 3500 mAh g1 for 20 cycles.
an initial reversible capacity of about 2200 mAh g1 with capacity
Moreover, the capacity of the Si NWs at faster rates was also
retention of 61.3% after 500 cycles. After annealing this sample for
excellent with the capacity remained at 2100 mAh g1 at 1 C rate
better interfacial adhesion between the Si thin film and the Cu
Hence, silicon nanowire battery electrode might make a truly
substrate, the capacity retention was further improved to 78.5% for
promising design, which could accommodate large strains without
500 cycles . Nevertheless, Si thin film encounter with the
pulverization, provide good electronic conduction, and display
trouble in production.
short lithium insertion distances. Similarly, 1D Si nanotubes, which
An advanced effective approach to improve the electrochemical
offers lower diffusion-induced stresses for the empty space inside,
performance of silicon anodes is to fabricate silicon-based 3D
show that the morphology of the nanotubes did not change after
composites with porous nanostructures, in which the local voidspace could partially accommodate the large volume change, thuspreventing the capacity from fading . A lotus-root-likemesoporous Si with carbon surface coating displayed a stablecapacity of ca. 1500 mAh g1 for 100 cycles at 1 C and a high ratecapability up to 15 C Macroporous silicon and carbon–siliconperiodic materials based on inverse-opal structures synthesizedvia templating with ordered colloidal spheres and subsequentsilicon deposition was demonstrated with high capacities at lowcurrents and decent capacity retentions, but their performance isseverely restricted due to the low electrical conductivity of silicon.
A capacity with carbon coated could be maintained above2100 mAh g1 for 145 cycles whereas the capacity of a siliconinverse-opal coated without amorphous carbon was completelylost by the 11th cycle Monodisperse porous silicon nano-spheres (MPSSs) were synthesized via hydrolysis process with
Fig. 1. Schematic of morphological changes that occur in Si during electrochemical
subsequent surface-protected magnesiothermic reduction. The Li-
cycling. NWs grown directly on the current collector do not pulverize or break into
ion battery (LIB) anodes based on MPSSs demonstrate a high
smaller particles after cycling. Rather, facile strain relaxation in the NWs allows
reversible capacity of 1500 mAh g1 after 500 cycles at C/2 .
them to increase in diameter and length without breaking. This NW anode design
Furthermore, nest-like Si nanospheres exhibit superior
has each NW connected with the current collector, allowing for efficient 1D elec-
lithium-storage capacity, high-rate capability and long cycling
tron transport down the length of every NW. The figure is reprinted from Ref. with permission.
properties as well.
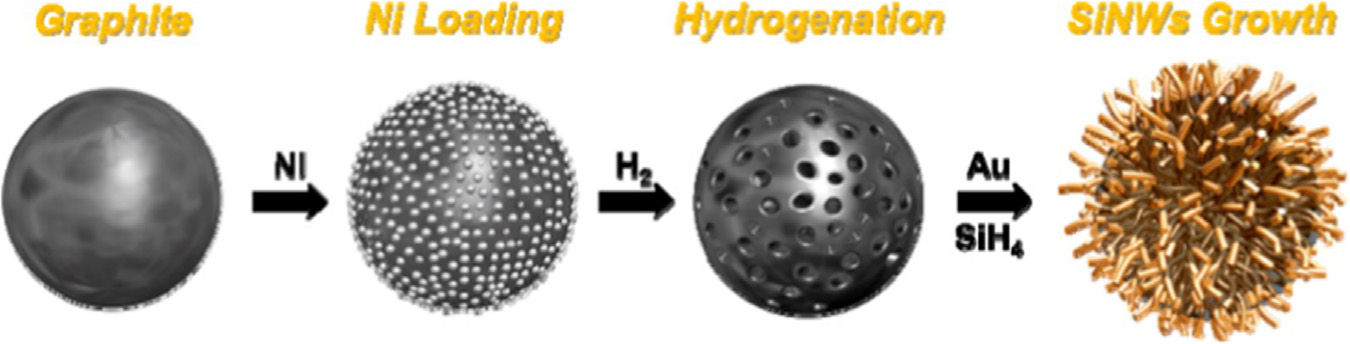
M. Zhang et al. / Energy Storage Materials 4 (2016) 1–14
From previous discussion, we find that even though large
carbon coated Si nanowires, nanotubes and porous structures in
volume change of pure Si could be accommodated by the rational
this method are usually high, ca. 2000 mAh g1 The
design of nano architectures, their cycling stability still much need
shortcomings of this method are bad operability, high cost and
to be further improved by the protection of carbon. Because the
poor scalability.
surface-to-volume ratio is high for these morphologies, the for-
Similarly, Si NPs could also deposited on commercial carbon
mation of stable SEI layers is less possible. At the same time, car-
structures, and these composites also show high capacities after
bonaceous materials normally could form stable SEI layers on their
long cycle numbers. For example, Yushin s group loaded Si NPs on
own surfaces. Thus, carbon coating might offer a good choice in
a 3D spherical carbon-black scaffold. The final Si/C nanohybrid
constructing long lifespan batteries for Si materials. Moreover, the
exhibited impressive electrochemical properties, including a high
incorporation of carbon can release stress of volume expansion
specific capacity above 1500 mAh g1 at a rate of 1 C after 100
contributing to long cycle life. Thus, recent progress for the roles of
cycles . An interesting architecture is Si nanowires internally
carbon in promoting the electrochemical performance of Si anode
grown from porous graphite, demonstrating a high volumetric
will be discussed more thoroughly in the following section.
capacity density of 1363 mAh cm3 with 91% Coulombic efficiencyand high rate capability of 568 mAh cm3 even at a 5 C rate.
3. The composite materials of nano silicon and carbon
A more cost efficient approach for preparing Si/C composite is
mixing Si with carbon or blending with carbon precursors with
Much effort has been not only put in preparing nanostructured
following pyrolysis process. For example, directly mixing Si pow-
silicon, but also come over constructing of nanohybrids with such
der with graphite, mesocarbon microbeads (MCMB) or hard car-
as metal and carbon for their ability to accommodate the volume
bon could achieve stable electrode morphology even after exten-
change and enhance electrical conductivity. Typical structures
ded cycling and the capacity retention could attain ca.
include metallic nanohybrids and carbonaceous nanohybrids.
500 mAh g1 after 400 cycles (for MCMB/Si mixture) Si can
There are some examples of applying Cu and Ag coatings that have
also blend with carbon sources by ball-milling and then pyrolyze
been shown to increase coulombic efficiency and improve rate
resulting mixture at high temperature . Silicon/carbon com-
capability. ,Metallic nanohybrids, due to a larger atomic
posites synthesized via the above methods often display reversible
density and higher cost, are practically unsuitable for the con-
capacities higher than graphite . When lithium metal was
struction of electron-transport pathways. As a result, a significant
added in previous of ball milling, lithiated silicon–carbon com-
amount of studies have focused on exploring lighter alternatives
posites display ca. 0.13% capacity loss per cycle with high specific
for the construction of similar electron-transport pathways in Si-
capacity ( 700 mAh g1) . Ternary composites such as flake
based anodes. The most common attempt is to combine the Si
graphite/silicon/carbon also show good cycling retention .
structure with a conducting carbonaceous layer, which possess
Various precursors can be used for encapsulation, including
many merits, such as excellent flexiblity, high conductivity, light-
resorcinol formaldehyde gel poly(vinylidenefluoride) (PVDF)
weight, electrochemical and thermal stability, in hoping to better
, polystyrene glucose , pitch , poly(vinyl chloride)
retain the integrity of Si particles and restrain active materials
(PVC) ,and so on. The hydrothermal method is another easy
disconnected from the conductive electrode. In this section, Si/
approach to homogeneously blend Si with precursors .
carbon composites are classified into two main classifications:
Spray pyrolysis, which could blend solvent type precursors toge-
(1) Si/traditional carbon (Si/TC) composites, including Si particles
ther and obtain powder product, was considered as an effective
with deposition carbon, pyrolytic carbon and commercial bulk
approach to synthesis various structures and composites with
carbons; (2) Si/nanocarbon (Si/NC) composites, including Si par-
good electrochemical performance . Spherical nanostructured
ticles with carbon nanotubes (CNTs), graphene and corresponding
Si/C composites could be prepared by spray drying technique with
3D architectures; and (3) Si/traditional carbon/nanocarbon (Si/TC/
phenol formaldehyde resin as the carbon source, which could
NC) composites. The Si/TC composites will be discussed first fol-
exhibit a relatively high reversible capacity and good cycle per-
lowed by Si/NC composites and Si/TC/NC composites.
formance used in lithium ion batteries Furthermore, spraydrying technique could be applicable for the large-scale produc-
3.1. Si/traditional carbon composites
tion of various Si/C composites .
There are some delicate designs prepared with special meaning
Si/traditional carbon (Si/TC) composites using traditional car-
but hard to classify. Here we will give a brief introduction of them.
bons will be discussed on the line of their preparation methods
A pioneer example is a yolk-shell structure design. This structure
and then some of the recent exciting and interesting design
was prepared through an intermediate sacrificial silica layer with
examples will be presented.
void space in between the Si particles and the carbon shell and
Conventionally, coating carbon on nano Si materials commonly
showed excellent capacity (2833 mAh g1 at C/10), long cycle life
adopted thermal decomposition or chemical vapor deposition
(1000 cycles with 74% capacity retention), and high coulombic
method with precursors of acetylene gas. These methods
efficiency (99.84%) Later, those hybrid nanoparticles are
result in carbon layers of high uniformity, neat surface smoothness
assembled into a thicker carbon layer. This microstructure could
and high purity. Thus, the initial capacity and capacity retention of
further lower the electrode-electrolyte contact area, resulting in
Fig. 2. Schematic view for the synthesis process of Si nanowires internally grown in porous graphite. The figure is reprinted from Ref. with permission.
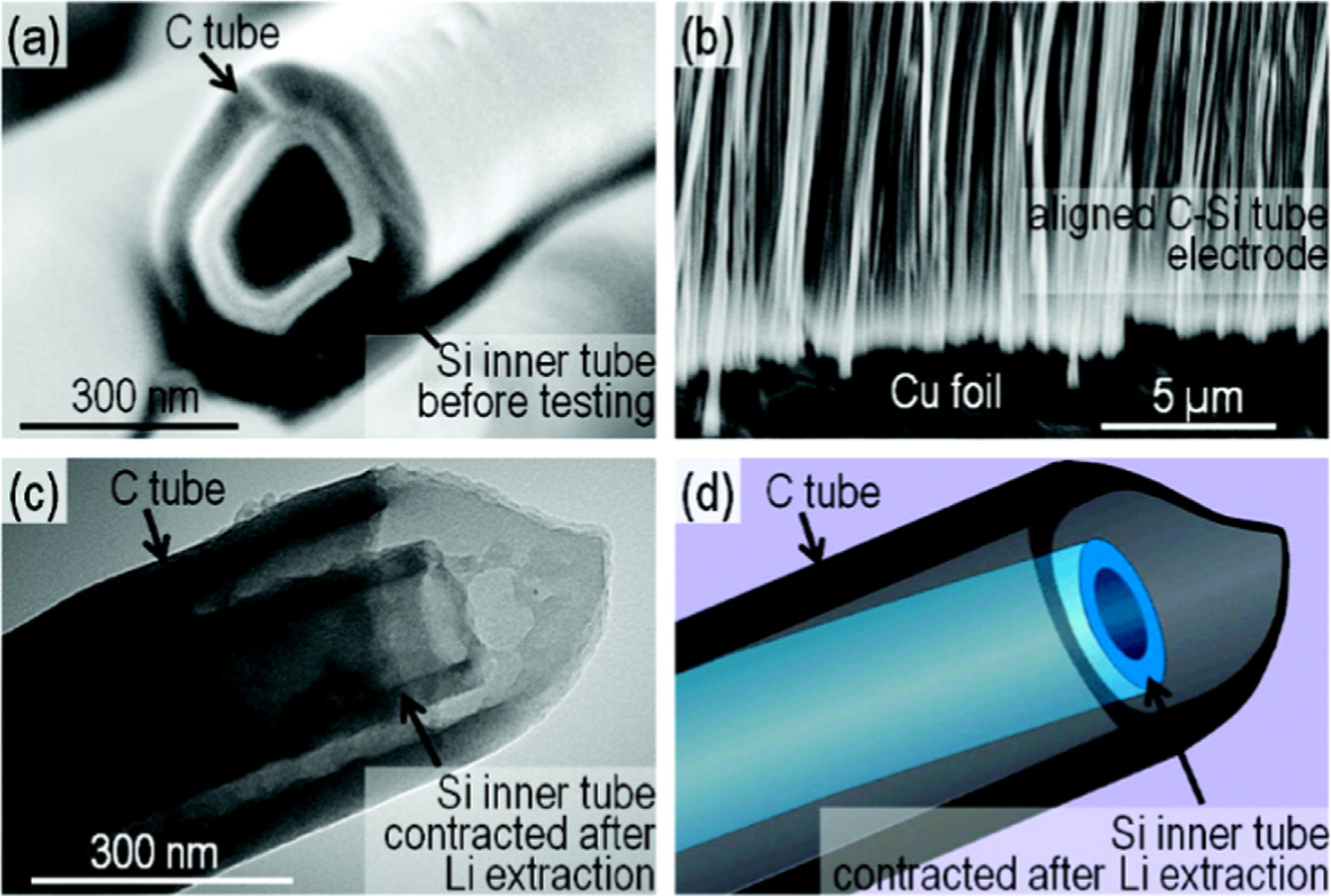
M. Zhang et al. / Energy Storage Materials 4 (2016) 1–14
higher Coulombic efficiency (99.87%) and volumetric capacity
simultaneously electrospraying nano-Si-PAN (polyacrylonitrile)
(1270 mAh cm3), and the cycling remains stable even when the
clusters and electrospinning PAN fibers followed by carbonization
areal capacity was increased to the level of commercial lithium-ion
with uniform incorporation of Si NPs into fiber paper. The flexible
batteries (3.7 mAh cm2)
3D Si/C fiber paper electrode demonstrated a very high overall
In short, Si/TC composites are very promising for LIB anodes.
capacity of 1600 mAh g1 with capacity loss less than 0.079% per
But the preparation of high quality carbon in easy and cost effi-
cycle for 600 cycles and excellent rate capability .
cient methods are still challenging.
The carbon nanofibers can not only serve as the conductive
matrix, but also encapsulate Si NPs into them. Here are two
3.2. Si/nanocarbon composites
interesting examples. Hwang et al. developed an electrospinningprocess to produce core–shell fiber electrodes with Si NPs as core
Nanocarbons such as fullerene carbon nanofibers (CNF)
wrapped in the carbon shell. This core–shell structure exhibited
–, carbon nanotubes (CNTs) and Graphene, intensively
high gravimetric capacity of 1384 mAh g1, excellent cycle life of
investigated for advanced energy storage with continuously gro-
300 cycles with almost no capacity loss . Later, Lee s group
wing academic and technological impetus, have been incorporated
demonstrated the almost full accommodation of all the volumetric
with Si NPs for the preparation of LIB anode materials
changes of Si by embedding Si NPs into a tunable cyclized-
Thus, the latest development for the composite anode materials of
polyacrylonitrile (cPAN) fiber network bonded together by a
Si with these nanocarbon materials, especially CNFs/CNTs and
Graphene, are discussed below.
3.2.2. Si/CNTs anode materials
3.2.1. Si/CNFs anode materials
Due to their excellent conductivity, high surface area,
One-dimensional (1-D) nanostructures have the advantages of
mechanical flexibility, and chemical stability CNTs, inclu-
high surface area and short ion diffusion length, and have been
ding single wall CNTs and multiwall CNTs , are considered as
viewed as components for next-generation electrochemical energy
promising materials to improve the performance of silicon anode
conversion and storage devices. The ductile CNF matrix can buffer
materials. There are many studies combining CNT with Si in
the Si volume expansion on the macro domain and maintain good
mainly two forms, considering the relative position of Si particles
contact with both the active materials and the electrolyte after
and CNTs: Si NPs on the inner walls of CNTs and Si NPs on the
lithium insertion and extraction cycles, resulting in high reversible
outer walls of CNTs
capacity and fairly good cyclability Sputter Si at CNF, the
When Si film was deposited on and weakly bonded to the inner
hybrid nanostructured Si/CNF anodes exhibited superior device
surface of a nano confined and size-preserving tubular material, Si
performance to materials used in previous studies, in terms of
deforms upon electrochemical alloying and dealloying without
both specific capacity and cycle life. The CNFs provide not only a
cracking. This model was verified by Yushin s group, who utilized
good strain/stress relaxation layer but also a conductive electron
carbon nanotubes (CNTs) with an inner Si coating. The composite
pathway . Vertically aligned carbon nanofiber (VACNF) provide
samples with a Si content of 46 wt% showed a capacity of
a good lithium-ion intercalation medium and a robust conductive
2100 mAh g1, very close to the theoretical maximum predicted,
core to effectively connect high-capacity silicon shells for lithium-
assuming Si's contribution to be 4200 mAh g1. Furthermore, a
ion storage. When VACNFs coaxially coated with silicon shells,
stable SEI layer on the carbon layer impermeable to solvent
an excellent cycle stability, about 89% of the capacity retention
molecules serves as a barrier to electrolyte decomposition and
after 100 charge–discharge cycles at the C/1 rate, has been
leads to a Coulombic efficiency 499.9% after the first cycle )
achieved Si/C fiber paper electrode could be synthesized by
Cheng s group revealed the confinement effect of CNTs
Fig. 3. Electron microscopy of the composite Si-in-C tubes: (a) scanning electron microscopy (SEM) of one of the synthesized samples, (b) SEM of the electrode attached to aCu current collector, (c) TEM and (d) its schematic of the sample after Li extraction at the 10th cycle. The figure is reprinted from Ref. with permission.
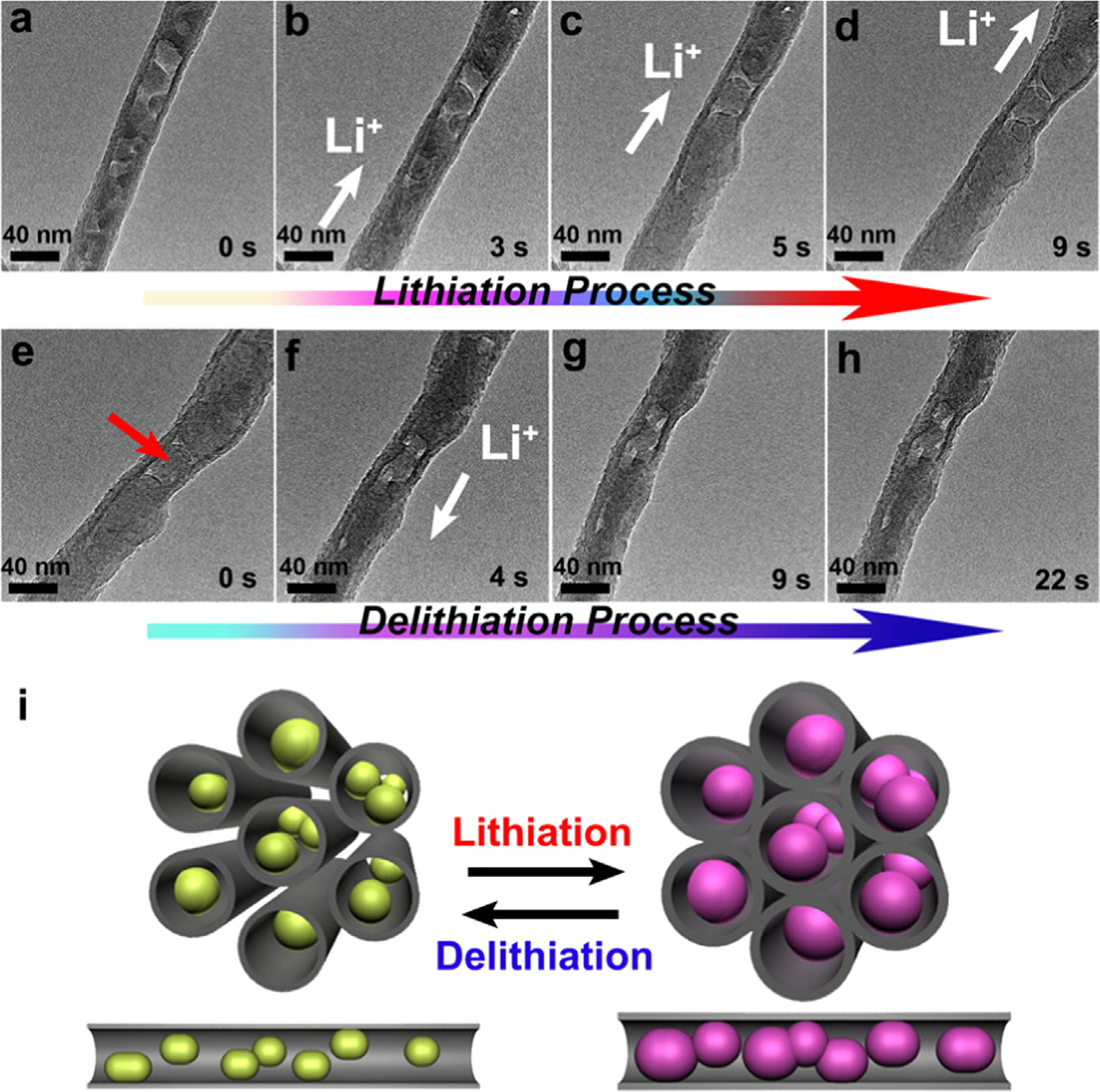
M. Zhang et al. / Energy Storage Materials 4 (2016) 1–14
when Si NPs encapsulated within the hollow cores of the CNTs.
3.2.3. Si/Graphene anode materials
The volume expansion of the lithiated Si NPs is restricted by the
Graphene plays important roles in electrode materials investi-
walls of the CNTs ()
gation, due to that it could provide a conductive channel for
As another combination method, Si NPs on the outer walls of
electron transport, optimize electrical contact between the elec-
CNTs take advantage of both the voids between the CNTs/N-CNTs
trode components and also act as a buffer to volume changes
and the tubular voids, thus effectively release the volume change
during cycling, which are all benefiting from the graphene's large
of Si Vertical aligned Si/CNTs composite can be prepared via
surface area, mechanical flexibility, chemical stability and excel-
a two-step CVD method: CVD growth of CNTs array on substrate
lent conductivity. Scientists have developed several methods to
and deposition of Si NPs on it –The covalent bonding of
fabricate composites of silicon nano particles/wires and graphene
CNTs to Si NPs can further enhance the electronic pathway to the
(Si/Graphene) composites for LIB recently.
active material particles and helps to prevent the detachment of Si
Graphene in Si/Graphene composites include the graphene
reduced from graphene oxide, exfoliated graphene and CVD
from CNTs upon repeated lithium insertion/extraction . The
growth of graphene among which reduced graphene oxide
capacity of the composite can reach 2000 mAh g1 with 0.15%
(RGO) is the most common used graphene. Si/Graphene compo-
decay per cycle in 25 cycles The gravimetric capacity is high,
sites can be developed through a simple facile way by filtering the
but volumetric (areal) capacity of Si/CNTs composite is relatively
silicon/graphene oxide solution to film with a following reduction
low. When the vertically aligned CNTs utilized as the conductive
treatment . The reversible specific capacity of this free
core and coated with pore size-graded Si film, this 4 μm thick
standing Si composites can reach 1500 mAh g1 after 200 cycles.
electrode deliver a high volumetric (areal) capacity and good cycle
Another interesting self-supporting binder-free silicon based
stability Volumetric (areal) capacity could also be increased
anode was prepared by double encapsulation of silicon nanowires
through preparing a multilayer Si/CNT coaxial by a layer-by-layer
(SiNWs) with two kinds of graphene (overlapping graphene
assembling technique .
(G) sheaths and reduced graphene oxide (RGO) overcoats) (
Generally speaking, Si/CNTs is a good candidate for LIB anodes
This resulted structure (SiNW@G@RGO) have a high rever-
and the encapsulation technique is an effective way to prevent
sible specific capacity of 1600 mAh g1 at 2.1 A g1, 80% capa-
pulverization and stabilize SEI layer, mainly because of the sig-
city retention after 100 cycles, and superior rate capability
nificantly enhanced conductivity and structural durability. Major
(500 mAh g1 at 8.4 A g1) .
concerns for this composite material are: (1) the complicated
Though both the filtration-directed assembly approach and sim-
fabrication process, which significantly increase the fabrication
ple mixing method have obtained improvements on lithium storage,
cost, and thus prevent its commercial application and (2) the loose
they do not provide good dispersion of Si NPs between graphene
binding between silicon and CNTs and the binding construction
sheets and good interfacial connection between Si NPs and graphene
between them is also expensive and time consuming.
sheets. An electrostatic attraction-directed self-assembly approach
Fig. 4. Dynamic structural changes of a Si NP-filled CNT under electrochemical lithiation/delithiation. (a-d) Lithiation of a Si NP-filled CNT. (e-h) Delithiation of the same SiNP-filled CNT. (i) Illustration of the lithiation/delithiation of Si NP-filled CNTs. The figure is reprinted from Ref. with permission.
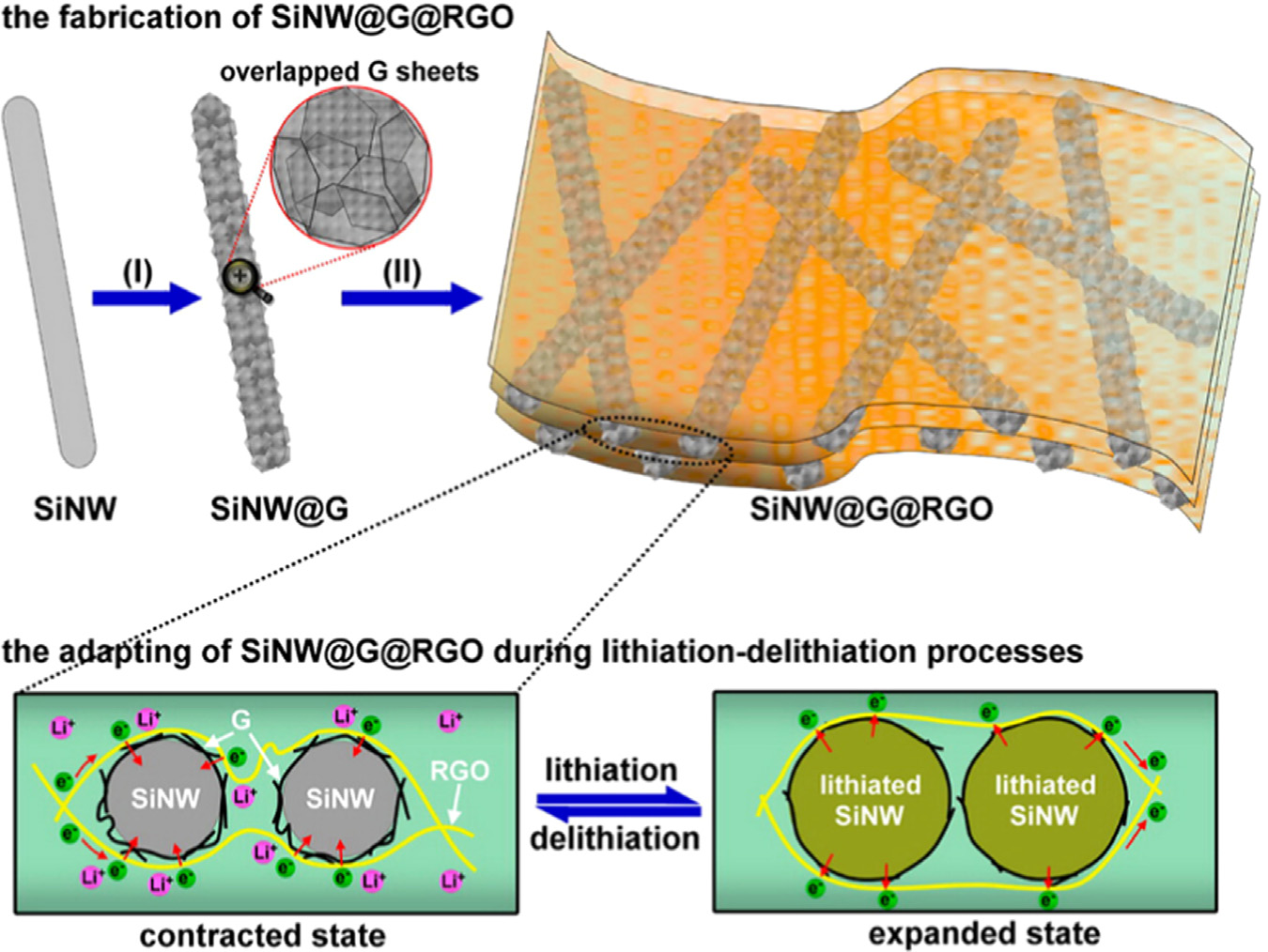
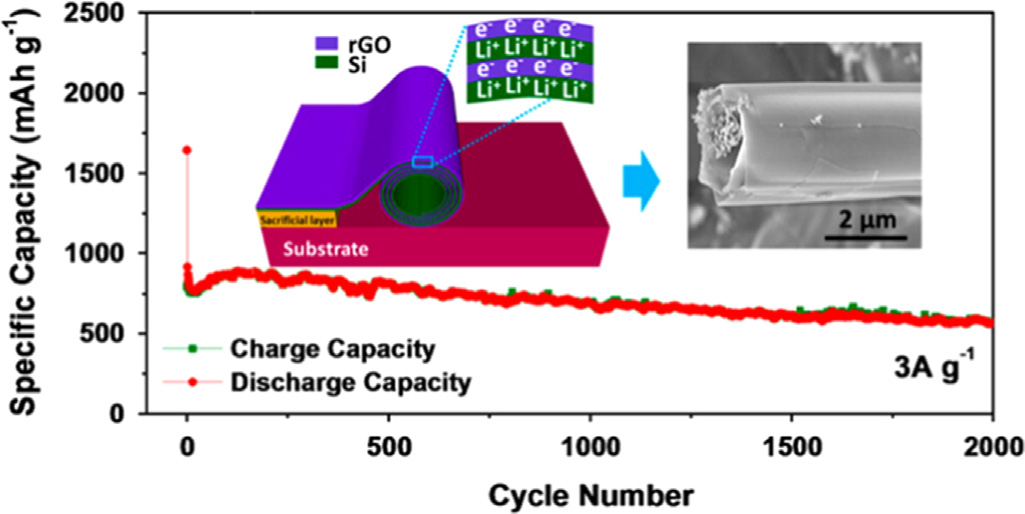
M. Zhang et al. / Energy Storage Materials 4 (2016) 1–14
Fig. 5. Schematic of the fabrication (upper panel) and adapting (lower panel) of SiNW@G@RGO. The fabrication process mainly includes (I) chemical vapor deposition (CVD)growth of overlapped graphene sheets on as-synthesized silicon nanowires (SiNWs) to form SiNW@G nanocables, and (II) vacuum filtration of an aqueous SiNW@G-graphene oxide (GO) dispersion followed by thermal reduction. The resulting SiNW@G@RGO can transform between an expanded state and a contracted state duringlithiation/delithiation cycles, thus enabling the stabilization of the silicon material. The figure is reprinted from Ref. with permission.
was developed with uniform dispersion of Si NPs on graphene sheetsThis approach realizes an uniform dispersion of Si NPs betweentwo layers of graphene sheets. The as-obtained composite exhibitsstable cycling performance (approximately 1205 mAh g1 after 150cycles) and excellent rate capability. The interfacial contact betweengraphene and other materials could be connected by mechanical orchemical interaction Dual chemical cross-linking and hydrogenbonding interactions between surface-modified Si NPs and grapheneoxide (GO) exhibited an outstanding capacity retention capabilityand good rate performance, delivering a reversible capacity of1000 mAh g1 after 400 cycles at a current of 420 mA g1 withalmost 100% capacity retention . Furthermore, Si wrapped ingraphene sheets can also be synthesized by a simple spray dryingprocess. This simple but effective nano/micro-assembly technology
Fig. 6. Schematic fabrication process of the rolled-up Si/rGO bilayer nanomem-branes. The figure is reprinted from Ref. with permission.
can be used for large-scale production of various graphene-basedcomposite materials with high performance for electrochemical
Si/RGO nanoarchitecture demonstrates long cycling life of 2000
energy storage and conversion .
cycles at 3 A g1 with a capacity degradation of only 3.3% per 100
To further improve the electrochemical properties of Si/Gra-
cycles. The inner void space inside the configuration together with
phene composites, works with delicate control of Si nanoparticle
the mechanical feature of the amorphous Si nano membranes can
size and coating graphene layers have been conducted. Si NPs
buffer the strain of lithiation/delithiation against pulverization to
downsized to ca. 3 nm silicon quantum dots and anchored on GNS,
extend cycling life. The alternatively aligned RGO layers in this
which exhibited an extraordinary rate capability due to the
nanostructure can facilitate electron transport, accommodate the
surface-controlled lithium storage behavior rather than conven-
volume change of Si layers and prevent their aggregation. Fur-
tional diffusion-controlled mechanisms . Another example is
thermore, the RGO layers can protect the nanomembranes from
graphene sheets of 2–10 layers directly grown on Si particles and
the excessive formation of thick SEI layer to suppress the capacity
the graphene layers rooted on the Si particles. This structure can
accommodate the large volume change of Si particle via a sliding
Si/Graphene composite is another good candidate for LIB
process between adjacent graphene layers. Thus, cells using the
anodes and the combination structure is effective to prevent the
above composite as anode could reach a high volumetric energy
mechanical pulverization, mainly because of the flexibility and
densities of 972 and 700 Wh L1 at first and 200th cycle, respec-
high specific area of graphene. However, the capacity is still
inevitably decaying, and the proposed reasons are as follows: (1)
The multilayer C/Si/C microtubes exhibited synergistic proper-
the low conductive connection between graphene and Si, espe-
ties and superior electrochemical performance when used as
cially during the lithium insertion and desertion process; (2) not
anodes for LIBs because its rolled-up layer by layer structure could
yet refined structural morphology of the composite.
buffer the strain of volume changes, and delay the pulverization of
Three-dimensional structures of active materials provide large
the electrode materials . When the carbon layer in the roll to
surface area, well defined pathways for the access of electrolyte
roll nanomembranes was replaced by RGO layer, the sandwiched
and mechanical stability for the integrity of electrodes .
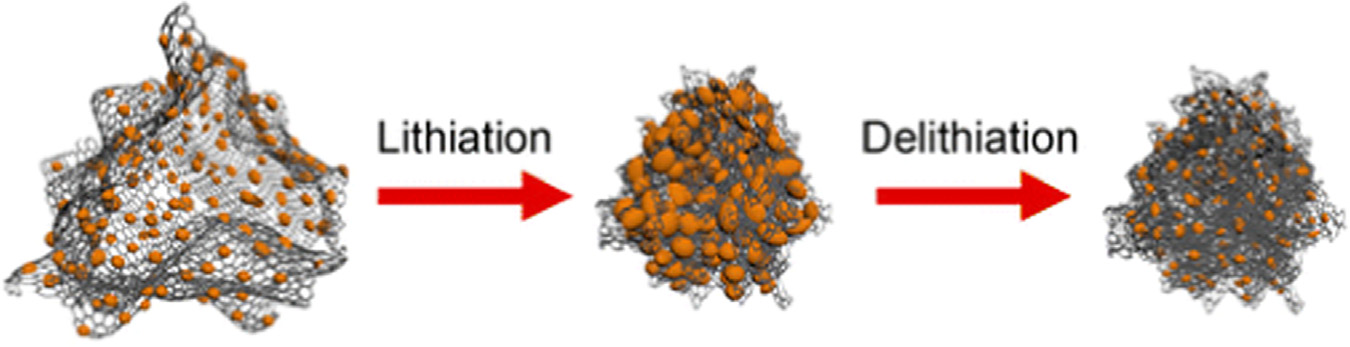
M. Zhang et al. / Energy Storage Materials 4 (2016) 1–14
Taking advantages of such extraordinary properties of graphene
carbon, encapsulating Si NPs, was anchored on the walls of RGO.
offers excellent opportunities for building attractive matrices,
Pyrolytic carbon plays three important roles in Si/TC/NC compo-
which not only accommodate the Si volume change, but also
site: (1) provide electrical conductivity; (2) reduce direct exposure
provide unobstructed electron and ion pathways.
of Si to electrolyte, thus decrease the unstable SEI growth; (3)
Si on 3D graphene framework composites could be prepared
serve as a glue to connect Si NPs with RGO. The RGO play key roles
through constructing 3D architectures, and then Si NPs or Si pre-
in: (1) provide good electrical contact and (2) provide large void
cursors being loaded on the as formed framework. The first
spaces to accommodate the volume change of Si. In the Si/Poly-
example is a hierarchical 3D mesoporous carbon-coated Si@gra-
mer/CNT composites, CNTs and PPy work together to improve
phene foam nanoarchitecture, which was prepared via magne-
conductivity and provide space for volume change . Si/Poly-
siothermic reduction of silica on the three dimensional matrix and
mer/CNT composites possess an advantage over Si/TC/RGO in that
exhibited superior electrochemical performance including a high
they do not need annealing at high temperature to recover the
lithium storage capacity of 1200 mAh g1 at the current density of
conductivity of RGO.
1 A g1 Another example is the amorphous Si NPs coated on
Furthermore, Silicon could also be well-anchored onto 3D NT/
backboned-graphene nanocomposite architecture. The electrode
TC frameworks. For example, Si @ 3D graphene/carbon nanotube
facilitates the electron transport and lithium diffusion, resulting in
(CNT)/ aerogels framework (CAs) nanohybrids could make use of
remarkable first-cycle Coloumbic efficiency of 92.5% with a high
both NT/TC layers and the balanced open voids, with a high
specific reversible capacity of 2858 mAh g1, excellent power
reversible capacity of 1011 mAh g1, and excellent capacity
capability, and outstanding cycling stability ) .
retention of 96% . However, the preparation method above
Compared with simple graphene sheets, which are easier to
was not satisfying for the complicate fabrication of 3D supporting
restack, hierarchical three-dimensional architectures could more
framework and deposition of Si. Therefore, some simple methods
effectively buffer the strain from the volume change of Si during
for the preparation of high performance Si on 3D network anode
the charging discharging process and preserve the high electrical
composites were also reported. Our group fabricated a ternary Si-
conductivity of the overall electrode, representing a new direction
based composite Si@C/GF through simple hydrothermal reaction
for fabricating robust, high-performance lithium-ion batteries and
and thermal reduction, in which Si NPs were coated by a thin
related energy storage applications with advanced nanostructured
carbon layer by pyrolysis of phenolic resin and encapsulated in a
graphene framework (GF). As a result, the double-protected Si NPshave a much improved cycle stability as well as high specific
3.3. Si/traditional carbon/nanocarbon composites
capacity and good rate performance . Another simple methodof preparation of 3D framework composite is Si NPs impregnated
Traditional carbon coating of nanocarbon/Si composite could
assemblies of templated carbon bridged oriented graphene, which
further effectively alleviate the aggregation of Si NPs by separating
was prepared by a modified vacuum filtration process followed by
them from each other and help nanocarbons build more efficient
thermal treatment (When used as LIB anodes, the 3D fra-
3D conducting networks. In the hybrids of graphene/carbon-
mework exhibited high gravimetric capacity (1390 mAh g1 at
coated Si NPs, the Si NPs are wrapped between graphene sheets
2 A g1 with respect to the total electrode weight), high volu-
and amorphous carbon coating layers. These two layers work
together to effectively suppress the aggregation and destruction of
(900 mAh g1 at 8 A g1) and excellent cyclic stability (0.025%
Si NPs, keeping the overall electrode highly conductive and active
decay per cycle over 200 cycles)
in Li storage Another graphene/Si–C hybrid (G/Si–C) is
We can summarize from , a‑Silicon @ backboned gra-
reported having a high areal capacity of 3.2 mAh cm2 after 100
phene nanocomposite (a-SBG) is the most effective approach to
cycles with high coulombic efficiency These findings
improve the performance of Si/C materials, for it show a high
demonstrate the importance of building a conductive network in
initial Coulombic efficiency of 92.5% and a high capacity of
the electrode level for efficient material utilization and may sug-
1103 mAh g1 at 14 A g1 after 1000 cycles with nearly 100%
gest future designs of Si-based anodes
capacity retention. However, the Si coating process is not com-
lists some combination works of Si NPs with TC and NC,
mercially viable for applications in large batteries using similar
in form of preparation methods and electrochemical properties, in
scalable technology. So the simple method of preparing 3D fra-
which Si/TC/RGO composites are more intensively investigated
mework composite with easy mixing, filtration and annealing
than Si/TC/CNT. This may be resulted from that GO, precursor of
process is attractive, and this composite has a relatively high initial
RGO, has functional groups and negatively charged that could
Coulombic efficiency of 72% and a high capacity of 1390 mAh g1
chemically bond or electrostatically connect with other carbon
at 2 A g1 after 200 cycles with 95% capacity retention. Simulta-
source or modified Si NPs. Most of Si/TC/RGO composite can be
neously, the in-situ polymerization of the mixture of Si, Py and
prepared via simple mixing of Si NPs with carbon source (carbon
CNT, and coating on the current collector for cell fabrication is also
sources are usually positive charged, serving as an intermedia
a good method to prepare the Si anode materials.
layer to electrostatically attract with negatively charged Si NPs and
In brief, Si NPs on frameworks of TC and NC is an excellent
GO) and GO, and then annealing at high temperature to pyrolysis
candidate for LIB anodes. The TC and NC framework could work
carbon source and reduce GO at the same time. Thus, the pyrolytic
together to provide both superior and robust conductivity and
Fig. 7. Schematic view of a-SBG nanocomposites before and after electrochemical cycling. The figure is reprinted from Ref. with permission.
M. Zhang et al. / Energy Storage Materials 4 (2016) 1–14
Table 1Electrical properties of Si anodes with Si/TC/NC composites.
Preparation method
Qr1 (mAh g1) (initial
Si @PANI @RGO, pyrolysis
Si&PANI&RGO, pyrolysis
PAN add into GO&Si, coat on Cu foil, pyrolysis
Si & PDA & GO mix – pyrolysis
Si & Py &CNT – in-situ polymerization – coat on Cu 70% (binder
Si wt%: mass loading of Si; Qr1: the first reversible capacity; CE: Coulombic efficiency; QdN(N): discharge capacity in Nth cycle; C.R.N.: capacity retention in Nth cycle. PANI:polyaniline; PDA: polydopamine; RF: resorcinol (R) and formaldehyde (F); PVP: polyvinylpyrrolidone; Py: Pyrrole.
Fig. 8. Electrode design and fabrication. (a) Schematic of the configuration of silicon nanoparticle-impregnated assemblies of templated carbon bridged oriented graphene(TCG-Si). (b) Schematic illustration showing the structure of TCG obtained by removing the Si template from the TCG-Si. (c) Schematic illustration of the fabrication processfor TCG-Si, where bovine serum albumin (BSA)-coated silicon nanoparticles and graphene oxide (GO) are assembled via electrostatic interactions during vacuum filtration,thus enabling the successful fabrication of TCG-Si. The figure is reprinted from Ref. with permission.
sufficient pores. Furthermore, the 3D framework could provide not
only superior conductivity but also sufficient pores for lithium ionpathways and volume change buffer. Thus, the construction of
The type of binder used in particulate electrodes could greatly
effective 3D TC/NC architecture with Si NPs encapsulated in it
influence the cycling lifetime. Poly(vinylidene) difluoride (PVDF) and
simultaneously and with low cost may be a research direction in
carboxymethylcellulose (CMC) are the most frequently used binder
in bulk powder electrodes, but these binders cannot sustain theelongation that occurs during volumetric expansion, leading to rapidcapacity fade . Poly(acrylic) acid (PAA) possessing
certain mechanical properties comparable to those of CMC but con-taining a higher concentration of carboxylic functional groups, has
It is noteworthy that the binders, electrolyte composition and
been shown to improve cycle life by enhancing the adhesion of
conductive additives also play important roles in improving the
electrode active materials to copper current collectors. Alginate, a
electrochemical performance of Si based LIB anodes besides the
natural polysaccharide extracted from brown algae, was introduced
morphology and composition of Si materials. Here, we will
as binder for Si NPs in LIBs and the capacity of Si NPs has been sig-
describe the noteworthy progress in these aspects.
nificantly improved to 2000 mAh g1 at a high charge rate of 1 C
M. Zhang et al. / Energy Storage Materials 4 (2016) 1–14
Table 2Preparation methods and electrical properties of Si anodes with Si disperse in 3D frameworks.
Preparation method
3D mesoporous silicon @ graphene
PU as template to get GF –TEOS load SiO2 – Mg
foam nanoarchitecture
reduction – C2H2 to carbon coating
a‑Silicon @ backboned graphene
Reduction of freeze-dried GO – decomposition of
nanocomposite (a-SBG)
Si @ 3D graphene/carbon nanotube
Polymerization of RF with CNT/G –freeze-dried
–decomposition of SiH4
Si&Py&CNT – in-situ polymerization – coat on Cu foil 70% (bin-
Nano Si 3D graphene-PF pyrolytic
HT – add P/F – HT –annealing
Si @ templated carbon-bridged
Si mixed with BSA – mixed with GO – filtration –
oriented graphene
Si wt%: mass loading of Si; Qr1: the first reversible capacity; CE: Coulombic efficiency; QdN(N): discharge capacity in Nth cycle; C.R.N.: capacity retention in Nth cycle; RF:resorcinol (R) and formaldehyde (F).
Fig. 9. Schematic illustration of 3D porous SiNP/conductive polymer hydrogel composite electrodes. Each SiNP is encapsulated within a conductive polymer surface coatingand is further connected to the highly porous hydrogel framework. The figure is reprinted from Ref. with permission.
. Although the linear, one dimensional (1D), backbones repre-
4.2. Electrolyte and electrode additives
sent a significant progress in the Si anode research, the multi-dimensional hyperbranched β-CD polymer with three dimensional
The incorporation of additives into electrolytes has been con-
backbones was reported to enhance Si-binder interactions as well as
sidered for enhancing the stability of the passivation layer because
improve mechanical stability of the electrode and therefore resolving
additives can alter the composition of SEI. Lithium bis(oxalato)
the chronic insufficient cycle lives of Si anode . After the concept
borate (LiBOB), fluoroethylene carbonate (FEC) and vinylene car-
of combining binding and conducting properties in the binder
bonate (VC), undergoing reductive decomposition at the silicon
surface at higher potentials than commonly-used ethylene car-
comethylbenzoic acid) (PFFOMB) binder as an example, self-
bonate (EC), is proposed to improve cycling stability by encoura-ging the formation of a stable SEI layer with a lowered resistance
healing polymers (SHPs), which can mechanically and electrically
for the diffusion of lithium ions on the surface of silicon electrodes.
heal cracks and damages, had been also demonstrated as effective
This avoids not only the decomposition of electrolyte but also the
binder to stabilize low-cost Si microparticle (SiMP) anodes. Com-
oxidation of the Si electrode As evidenced by the dif-
pared with traditional polymer binders, the self-healing chemistry is
ference of SEI layer of Si film anode formed in VC-free and VC-
designed to enable spontaneous repair of the mechanical damage in
containing electrolytes, the SEI layer formed in VC-containing
the electrode and thus increase the lifetime of the SiMP anode
electrolyte possessed better properties, which was impermeable
In-situ polymerization of conducting polymer hydrogel is
to electrolyte and the impedance kept almost invariant upon
another effective novel binder conception, which forms a continuous
cycling. In addition, SEM imaging revealed that the electrode
three-dimensional (3D) pathway for electronic conduction and pro-
cycled with VC had a smooth surface with small cracks believed to
vide sufficient voids for the expansion of Si () . By taking
form during delithiation, compared to a much rougher surface
advantage of the conductive polymer matrix, which provides fast
with protruding crystallites and an inhomogeneous appearance
electronic and ionic transfer channels, as well as free space for Si
without VC Detailed investigations are needed to guide our
volume changes, the electrode could be continuously deeply cycled
further advancement of electrolyte additives.
up to 5000 times without significant capacity decay. The following
The choice of the conductive additive and the quantities of
research could concentrate on the fabrication of binders with high
additive added into the electrode can have a significant impact on
mechanical strength, excellent electrical conductivity and more
electrochemical performance. The addition of carbon nanotubes
importantly, simple preparation process with low cost.
and reduced graphene, which have larger theoretical surface area
M. Zhang et al. / Energy Storage Materials 4 (2016) 1–14
compared to the active material (such as super P and acetylene
black), were shown to enhance both capacity and cycling stability
by forming a more efficient percolation pathway compared that
when larger later active additive particles were used . It
is also found that the amount of conductive additive can have a
profound effect on the cycle life of the electrode, which increases
with increasing conductive additive content For example, Si
NPs with a mean diameter of 78 nm that were mixed with carbon
black in a 1:1 weight ratio showed a reversible capacity above
1700 mAh g1 over ten cycles To obtain high energy density
in practice, addition of less advanced additive and attain better
electrochemical performance is attractive.
5. Conclusion and perspective
The discussion in this review has illustrated that significant
amount of researches are devoted into overcoming the challenges
of using silicon anodes in practical lithium ion batteries. Rational
designs of nanoparticles are effective to accommodate the large
volume change, but they are relatively expensive and must
incorporate with carbon to attend acceptable capacity retention.
Si/C composite could not only address the volume problems but
also help to stabilize the SEI layers. Nano carbons features their
remarkable properties and are more attractive in constructing of Si
composite to further improve the cycling stability. Battery com-
posites other than electrode materials, such as electrolyte addi-
tives and conductive additives, are also play important roles in
improving the cycle stability of Si anodes.
Even though considerable improvements has been achieved,
future research is still necessary along the following directions for
their practical application in commercial LIBs: (1) To the electrode
material aspect: we should explore more structurally and com-
positionally complex hierarchical composite nanostructures with
not only internal void/pore space to buffer the large volume
change but also good conductivity to attend high capacity reten-
tion during long cycle life; the manufacture process of those
nanomaterials should be simple and liable and the cost of raw
nanomaterials should be also accessible. (2) To other aspects: new
low cost, effective binders, electrode and electrolyte additives are
needed to maintain efficient electronic and ionic conduction from
silicon to the current collectors and stabilize the SEI layers during
cycling. (3) To practical fabrication aspect: the scalability, manu-
facturability, and cost of the nanomaterials are crucially important
to the eventual success in the practical applications. Furthermore,
the volumetric capacity, as an important parameter, should be
given more attention in future research and practical manufacture.
The authors gratefully acknowledge financial support from the
MOST (Grants 2012CB933401 and 2014CB643502); NSFC (Grants
51273093, 21374050, 51373078, 51422304 and 51472124); NSF of
Tianjin City (Grant 13RCGFGX01121); Collaborative Innovation
Center of Chemical Science and Engineering (Tianjin).
M. Zhang et al. / Energy Storage Materials 4 (2016) 1–14
M. Zhang et al. / Energy Storage Materials 4 (2016) 1–14
M. Zhang et al. / Energy Storage Materials 4 (2016) 1–14
Source: http://nanocenter.nankai.edu.cn/PDF/Energy%20Storage%20Materials_2016_ZM.pdf
ARTROSCOPIA VOL. 19, Nº 4 : 161-167 2012 Eficacia de la Dexametasona como Coadyuvante en Bloqueo Interescalénico Dr. Luciano Deganutti,1 Dr. Rodrigo Carrizo,1 Dr. Fabián Astore,2 Dr. Pablo Narbona3 1Residente de Anestesiología, 2 Jefe de servicio de Anestesiología,3Médico Traumatólogo, especialista en artroscopía de hombro; Sanatorio Allende Córdoba
COMITÉ DE EVALUACIÓN DE NUEVOS MEDICAMENTOS DE ARAGÓN INFORME DE EVALUACIÓN Principio activo LINAGLIPTINA / METFORMINA Nombre comercial y presentaciones recubiertos con película (Boehringer Ingelheim Internacional GmbH) (65,57 €) ▼ JENTADUETO® 2,5mg/850mg 60 comprimidos recubiertos con película (Boehringer Ingelheim Internacional GmbH) (65,57 €)