Weifengxu.mit.edu
L-Type Calcium Channels: The Low Down
Diane Lipscombe, Thomas D. Helton and Weifeng Xu
J Neurophysiol
92:2633-2641, 2004.
You might find this additional info useful.
This article cites 135 articles, 77 of which can be accessed free at:
This article has been cited by 60 other HighWire hosted articles, the first 5 are:
Insulinotropic effect of high potassium concentration beyond plasma membrane
depolarization
M. Belz, M. Willenborg, N. Görgler, A. Hamada, K. Schumacher and I. Rustenbeck
, March 15, 2014; 306 (6): E697-E706.
Am J Physiol Endocrinol Metab
Ion channels in the endometrium: regulation of endometrial receptivity and embryo
implantation
Ye Chun Ruan, Hui Chen and Hsiao Chang Chan
Hum. Reprod. Update, March 2, 2014; .
Mechanisms of retroaxonal barrage firing in hippocampal interneurons
Mark E. J. Sheffield, Gabrielle B. Edgerton, Robert J. Heuermann, Tara Deemyad, Brett D.
Mensh and Nelson Spruston
J Physiol, October 1, 2013; 591 (19): 4793-4805.
Modulation of calcium currents and membrane properties by substance P in the lamprey
spinal cord
Carolina Thörn Pérez, Russell H. Hill and Sten Grillner
J Neurophysiol, July 15, 2013; 110 (2): 286-296.
Calcium dynamics during NMDA-induced membrane potential oscillations in lamprey
spinal neurons
contribution of L-type calcium channels (Ca
Di Wang, Sten Grillner and Peter Wallén
J Physiol, May 15, 2013; 591 (10): 2509-2521.
including high resolution figures, can be found at:
Updated information and services
Additional material and information
Journal of Neurophysiology can be found at:
This information is current as of May 8, 2014.
Journal of Neurophysiology publishes original articles on the function of the nervous system. It is published 12 times a year
(monthly) by the American Physiological Society, 9650 Rockville Pike, Bethesda MD 20814-3991. Copyright 2004 by the
American Physiological Society. ISSN: 0022-3077, ESSN: 1522-1598. Visit our website at http://www.the-aps.org/.
J Neurophysiol 92: 2633–2641, 2004;10.1152/jn.00486.2004.
L-Type Calcium Channels: The Low Down
Diane Lipscombe, Thomas D. Helton, and Weifeng Xu
Department of Neuroscience, Brown University, Providence, Rhode Island 02912
Submitted 9 May 2004; accepted in final form 5 June 2004
Lipscombe, Diane, Thomas D. Helton, and Weifeng Xu. L-type
agonists and antagonists has proved critical for their identifi-
calcium channels: The low down.
J Neurophysiol 92: 2633–2641,
cation in physiological assays and also for their biochemical
2004; 10.1152/jn.00486.2004. L-type calcium channels couple mem-
isolation (Kanngiesser et al. 1988). Biochemical purification of
brane depolarization in neurons to numerous processes including gene
the dihydropyridine receptor from skeletal muscle was the
expression, synaptic efficacy, and cell survival. To establish the
essential step in cloning the first voltage-gated calcium channel
contribution of L-type calcium channels to various signaling cascades,
(Tanabe et al. 1987). Sequence information from this landmark
investigators have relied on their unique pharmacological sensitivityto dihydropyridines. The traditional view of dihydropyridine-sensitive
study was then used to screen for and clone Ca 1.2 and Ca 1.3
L-type calcium channels is that they are high-voltage–activating and
cDNAs (Biel et al. 1990; Hui et al. 1991; Koch et al. 1990;
have slow activation kinetics. These properties limit the involvement
Mikami et al. 1989; Perez-Reyes et al. 1990; Williams et al.
of L-type calcium channels to neuronal functions triggered by strong
1992). Functional analyses of cloned channels, primarily of
and sustained depolarizations. This review highlights literature, both
Ca 1.2, were generally consistent with native cardiac L-type
long-standing and recent, that points to significant functional diversity
channels and the following criteria evolved for their identifi-
among L-type calcium channels expressed in neurons and other
excitable cells. Past literature contains several reports of low-voltage–
1) Activation by strong depolarizations (high-voltage–acti-
activated neuronal L-type calcium channels that parallel the unique
vated [HVA]).
properties of recently cloned Ca 1.3 L-type channels. The fast kinet-
2) High sensitivity to dihydropyridine agonists and antago-
ics and low activation thresholds of Ca 1.3 channels stand in stark
contrast to criteria currently used to describe L-type calcium channels.
A more accurate view of neuronal L-type calcium channels encom-
3) Relatively slow activation kinetics.
passes a broad range of activation thresholds and recognizes their
4) Calcium-dependent inactivation with little voltage-de-
potential contribution to signaling cascades triggered by subthreshold
pendent inactivation (
long-lasting).
5) Large single-channel conductance.
However, a substantial body of evidence points to hetero-
geneity among neuronal L-type calcium channels that has until
L-type calcium channels regulate numerous
recently received little attention. This review highlights recent
studies of cloned channels, as well as long-standing studies of
L-type calcium channels are perhaps the best characterized
native L-type channels, that point to significant deviations from
of the voltage-gated calcium channels. They were first recog-
criteria listed above in the properties of L-type channels.
nized as essential for coupling excitation to contraction inskeletal, cardiac, and smooth muscle cells (Beam et al. 1989;
Ca 1 genes encode L-type calcium channels
Franzini-Armstrong and Protasi 1997; Reuter 1985; Schneider
and Chandler 1973; Tanabe et al. 1990). L-type calcium
Identifying the genes that encode core Ca ␣ subunits of
channels are also expressed in neurons and endocrine cells
voltage-gated calcium complexes has led to a comprehensive
where they regulate a multitude of processes including secre-
sequence-based classification scheme (Fig. 1). When se-
tion of neurohormones and transmitters, gene expression,
quences are compared, voltage-gated calcium channels fall into
mRNA stability, neuronal survival, ischemic-induced axonal
three main groups: Ca 1 (L-type), Ca 2 (P-type, N-type, and
injury, synaptic efficacy, and the activity of other ion channels
R-type), and Ca 3 (T-type) (Ertel et al. 2000; Lipscombe
(Ashcroft et al. 1994; Bading et al. 1993; Bean 1989; Charles
2002b). In general, these gene families correspond to the
et al. 1999; Christie et al. 1997; De Koninck and Cooper 1995;
subtypes of calcium channels defined by functional and phar-
Deisseroth et al. 1998; Dunlap et al. 1995; Finkbeiner and
macological criteria. Ca 1 and Ca 2 genes are more closely
Greenberg 1998; Fuchs 1996; Galli et al. 1995; Heidelberger
related to each other when compared with Ca 3 genes. Ca 3
and Matthews 1992; Kamsler and Segal 2003; Lei et al. 2003;
T-type channels possess certain functional properties that set
Marrion and Tavalin 1998; Marshall et al. 2003; Murphy et al.
them apart from other voltage-gated calcium channels (Perez-
1991; Norris et al. 1998; Ouardouz et al. 2003; Sand et al.
Reyes et al. 1998). Low-voltage–activating calcium current
2001; Schorge et al. 1999; Shinnick-Gallagher et al. 2003;
typically marks the presence of Ca 3 T-type channels. How-
Smith et al. 1993; Tachibana et al. 1993; Thaler et al. 2001;
ever, as we will discuss, this property is shared by Ca 1.3, a
Thibault et al. 2001; Weisskopf et al. 1999; Wiser et al. 1999;
member of the Ca 1 gene family, and thus should not be
Zhang and Townes-Anderson 2002). The unique pharmacolog-
considered unique to Ca 3 (Avery and Johnston 1996; Kos-
ical sensitivity of L-type calcium channels to dihydropyridine
chak et al. 2001; Lipscombe 2002a; Platzer et al. 2000; Scholze
Address for reprint requests and other correspondence: D. Lipscombe,
The costs of publication of this article were defrayed in part by the payment
Department of Neuroscience, Brown University, 190 Thayer Street, Provi-
of page charges. The article must therefore be hereby marked "
advertisement"
dence, RI 02912 (E-mail:
[email protected]).
in accordance with 18 U.S.C. Section 1734 solely to indicate this fact.
0022-3077/04 $5.00 Copyright 2004 The American Physiological Society
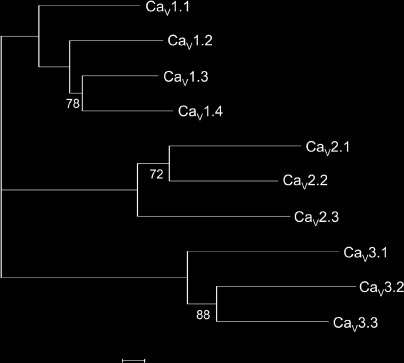
D. LIPSCOMBE, T. D. HELTON, AND W. XU
Schultz et al. 1993; Soldatov 1992; Takimoto et al. 1997;Welling et al. 1997). This channel opens as the membranepotential depolarizes beyond about ⫺30 mV. Ca 1.2 channels
help define the shape of the action potential in cardiac andsmooth muscle. These channels function primarily as calciumion channels and, unlike Ca 1.1 of skeletal muscle, calcium
flow through Ca 1.2 is an essential step in initiating the
signaling cascade that leads to cardiac and smooth musclecontraction (Reuter et al. 1988; Tanabe et al. 1990). In neuronsCa 1.2 channels are thought to couple membrane depolariza-
tion to regulation of gene expression (Dolmetsch et al. 2001;Weick et al. 2003).
The Ca 1.3 gene, formerly ␣ , is expressed in many of the
same cells that express Ca 1.2. In neurons, Ca 1.2 and Ca 1.3
are often found in the same general neuronal compartments,particularly dendrites, but their subcellular distributions appear
Ca ␣ subunit gene tree. Full-length amino acid sequences for all
distinct (Hell et al. 1993; Westenbroek et al. 1998). Ca 1.3 has
10 human Ca ␣ genes were aligned using a branch and bound tree search with
been found to co-localize with the small conductance calcium-
maximum parsimony (Genetic Computer Group, paupsearch and paupdisplay
activated potassium channel (Bowden et al. 2001). Ca 1.3 is
programs). Accession numbers for sequences used are: Ca 1.1, L33795;
Ca 1.2, AJ224873; Ca 1.3, M76558; Ca 1.4, AJ224874; Ca 2.1, AB035727;
also expressed in pancreatic beta cells, neuroendocrine cells,
Ca 2.2, M94173; Ca 2.3, L27745; Ca 3.1, AF190860; Ca 3.2, AF05196;
photoreceptors, amacrine cells, and hair cells of the inner ear
Ca 3.3, AF211189. Confidence values for each node were determined by
where it mediates synaptic transmission (Habermann et al.
bootstrap analysis. All unlabeled nodes represent 100% confidence. Represen-
2003; Ihara et al. 1995; Kollmar et al. 1997a,b; Liu et al. 2004;
tation was rooted using the midpoint method. Scale bar represents 1 substitu-tion per 100 amino acids. This tree is essentially the same when we exclude the
Morgans 1999; Morgans et al. 1998; Platzer et al. 2000; Russo
variable intracellular loops (I–II, II–III) and N- and C-termini (Lipscombe
et al. 2003; Safa et al. 2001; Scholze et al. 2001; Seino et al.
2002b). Our analysis indicates a stronger similarity between Ca 1.3 and
1992; Taylor and Morgans 1998). In the heart, Ca 1.3 is
Ca 1.4 genes compared with that of other published Ca gene trees (Ertel et
present in atrial tissue where it contributes to pacemaking
al. 2000). If we just align sequences of the 4 Ca 1 genes the confidence value
(Mangoni et al. 2003; Platzer et al. 2000; Takimoto et al. 1997;
at the Ca 1.3–Ca 1.4 node increases to 100%.
Zhang et al. 2002), but not in ventricular muscle that expresses
et al. 2001; Xu and Lipscombe 2001). Until recently, all
members of Ca 1 and Ca 2 gene families were considered
The Ca 1.4 gene, formerly ␣ , is expressed primarily in
high-voltage–activated. Ca 1 channels are distinguishable
retina and is linked to a rare human disorder, stationary night
from Ca 2 channels primarily by their unique pharmacology.
blindness (Bech-Hansen et al. 1998; Strom et al. 1998). Ca 1.4
Ca 1 channels are sensitive to dihydropyridine agonists and
is found at synaptic terminals of retinal bipolar cells, and RNA
antagonists, but are not blocked by -aga IVA or -conotoxin
encoding Ca 1.4 has also been PCR amplified from dorsal root
GVIA, which inhibit Ca 2.1 and Ca 2.2 channels, respectively
ganglia (Berntson et al. 2003; Murakami et al. 2001). Interest-
(Bean 1991; Cruz et al. 1987; McIntosh et al. 1999; Mintz et al.
ingly, the Ca 1.4 gene sequence is more homologous to
1992). However, as we will discuss, dihydropyridine antago-
Ca 1.3, based on comparisons among available Ca
nists do not completely inhibit all L-type channels.
Four mammalian Ca 1 genes encode L-type
L-type calcium channels are functionally diverse
calcium channels
Analyses of Ca 1 clones in various heterologous expression
Four Ca 1 genes are present in the human genome, referred
systems have provided compelling data that L-type calcium
to as Ca 1.1–1.4 (Fig. 1). The Ca 1.1 gene, formerly ␣ , is
channels are a functionally heterogeneous family.
expressed in skeletal muscle. Ca 1.1 directly links to ryano-
1) Not all L-type calcium channels require strong depolar-
dine receptors in the sarcoplasmic reticulum (Flucher and
izations for activation; Ca 1.3 and Ca 1.4 channels have low
Franzini-Armstrong 1996). Ca 1.1 primarily acts as a voltage
sensor, coupling depolarization to release of intracellular cal-
2) L-type calcium channels are not all inhibited equally well
cium by activating the ryanodine receptor. The influx of
by dihydropyridine antagonists; Ca 1.3 and Ca 1.4 L-type
calcium through the ion pore of Ca 1.1 during gating is
channels are significantly less sensitive compared with Ca 1.2.
secondary to its primary role as a voltage sensor (Schwartz et
3) Activation kinetics of L-type calcium channels vary.
al. 1985). The coupling between depolarization and channel
Ca 1.3 channels activate with fast kinetics, whereas Ca 1.1
opening is inefficient; Ca 1.1 channels open with slow kinetics
channels open slowly.
(Almers and Palade 1981; Rios and Brum 1987; Tanabe et al.
4) Certain Ca 1.4 L-type channels do not exhibit calcium-
dependent inactivation. Furthermore, in physiological solu-
The Ca 1.2 gene, formerly ␣ , is expressed in a variety of
tions that contain calcium, L-type calcium channels that do
cells including ventricular cardiac muscle, smooth muscle,
undergo calcium-dependent inactivation are not long lasting.
pancreatic cells, fibroblasts, and neurons (Diebold et al. 1992;
5) Most L-type calcium channels have relatively large sin-
Koch et al. 1990; Mori et al. 1993; Perez-Reyes et al. 1990;
gle-channel conductances when isotonic barium is the charge
J Neurophysiol • VOL 92 • NOVEMBER 2004 • www.jn.org
L-TYPE CALCIUM CHANNELS
carrier. However, analyses of single Ca 1.3 and Ca 1.4 chan-
The Ca 1.3 knockout mice, however, renewed interest and
nels are lacking.
provided compelling evidence that the Ca 1.3 gene encodes
L-type calcium channels with unusual properties (Mangoni et
Skeletal muscle Ca 1.1 and cardiac Ca 1.2 L-type channels
al. 2003; Platzer et al. 2000; Zhang 2002). At the behavioral
are functionally distinct
level, mice lacking the L-type Ca 1.3 gene experience signif-
icant sinoatrial node dysfunction characterized by sinus brady-
Although the first Ca ␣ subunit to be cloned, Ca 1.1
cardia. This unanticipated role for Ca 1.3 in pacemaking
resisted functional reconstitution in nonmuscle, heterologous
implies that these L-type calcium channels are important in
expression systems (Tanabe et al. 1987). Successful expression
mediating subthreshold depolarizations in the sinoatrial node.
of Ca 1.1 was eventually achieved using embryonic muscle
The absence of a low-threshold activating calcium current in
from dysgenic mice. In a series of classic experiments, Numa,
sinoatrial node cells of Ca 1.3 ⫺/⫺ mice confirmed this
Beam, and colleagues, rescued excitation– contraction cou-
hypothesis (Zhang 2002). Hearing loss and the absence of a
pling as well as L-type calcium channel currents in dysgenic
low-threshold activating calcium current in hair cells from
muscle by expressing Ca 1.1 cDNA in these cells (Adams et
these mice are consistent with prominent expression of Ca 1.3
al. 1990; Tanabe et al. 1988). Ca 1.1 currents were small and
in inner hair cells of the cochlea (Kollmar et al. 1997b; Platzer
activated with slow kinetics, properties consistent with native
et al. 2000). The functional properties of Ca 1.3 clones iso-
L-type currents in skeletal muscle. As in skeletal muscle,
lated from neurons and endocrine cells, more recently, confirm
contraction depended on the mobilization of intracellular cal-
that Ca 1.3 L-type channels activate at subthreshold voltages
cium stores. Ca 1.2 could also reconstitute excitation– contrac-
(Koschak et al. 2001; Platzer et al. 2000; Safa et al. 2001; Xu
tion coupling in dysgenic muscle, but the features were dis-
and Lipscombe 2001).
tinctly cardiac-like. In this case, calcium flux across the mem-brane through Ca 1.2 channels was essential to trigger muscle
Ca 1.3 L-type channels activate at relatively hyperpolarized
contraction (Tanabe et al. 1990). Ca 1.2 channels opened with
rates that were significantly faster compared with Ca 1.1.
Chimeric analyses demonstrated that sequence differences in
Figure 2 illustrates that Ca 1.2 and Ca 1.3 channels have
the II–III intracellular linker region of Ca 1.1 and Ca 1.2
very different activation thresholds. The Ca 1.2 and Ca 1.3
genes imparted the skeletal or cardiac muscle form of excita-
clones used were isolated from neuronal tissue, expressed in
tion– contraction coupling. In subsequent experiments Beam
tsA201 cells, and recorded under identical conditions. With
and colleagues demonstrated that sequence differences in the
physiological concentrations of extracellular calcium, Ca 1.3
domain IS3–IS4 linkers of Ca 1.1 and Ca 1.2 genes deter-
channels start to activate at about ⫺55 mV, a voltage that is
mined the gating phenotypes of these two L-type calcium
approximately 20 –25 mV more hyperpolarized as compared
channels (Nakai et al. 1994). The slow gating phenotype of
with Ca 1.2. Low-threshold activation is a prominent feature
Ca 1.1 channels was transferred to Ca 1.2 by swapping in the
of all Ca 1.3 clones isolated recently, independent of tissue of
IS3–IS4 linker of Ca 1.1. Variations in S3–S4 linker se-
origin and of auxiliary subunits (Koschak et al. 2001; Safa et
quences among other voltage-gated ion channel gene families
al. 2001; Scholze et al. 2001; Xu and Lipscombe 2001). Why
and their splice isoforms are similarly important in modulating
was this unique and salient feature of Ca 1.3 not highlighted in
channel gating kinetics (Lipscombe 2002b; Mathur et al. 1997;
earlier studies (Bell et al. 2001; Ihara et al. 1995; Williams et
Tang and Papazian 1997).
al. 1992)? The most likely explanation relates to the use of highconcentrations of extracellular barium and calcium in these
Ca 1.2 and Ca 1.3 L-type channels are functionally distinct
studies to compensate for low expression levels. Under these
conditions, Ca 1.3 channels would have activated at signifi-
With the exception of skeletal muscle and perhaps retina, all
cantly more depolarized voltages as a result of surface charge
excitable cells express one or both Ca 1.2 and Ca 1.3 genes.
screening (Frankenhaeuser and Hodgkin 1957; Hille 2001).
The products of these genes constitute the major fraction of
Indeed, when we studied Ca 1.3 L-type currents under similar
L-type calcium channels in mammals (Hell et al. 1993; Ludwig
conditions, 40 mM extracellular barium, the current–voltage
et al. 1997; Takimoto et al. 1997; Williams et al. 1992). Until
relationship shifted into the range of a high-voltage–activated
recently, the prevailing image of the neuronal L-type calcium
L-type calcium channel (Xu and Lipscombe 2001). Additional
channel was of a high-voltage-activated, slowly activating
factors such as interactions with other subunits, modulation by
channel with high sensitivity to dihydropyridines (Ertel et al.
second-messenger signaling cascades, and alternative splicing
2000; Hille 2001). These features have developed primarily
have the potential to influence channel properties (Birnbaumer
from biophysical analyses of heterologously expressed Ca 1.2
et al. 1998; Lipscombe 2002b; Scholze et al. 2001). However,
L-type calcium channels (Altier et al. 2001; Bourinet et al.
low-voltage activation appears to be a salient feature of
1994; Charnet et al. 1994; de Leon et al. 1995; Ivanina et al.
Ca 1.3-containing channels (Koschak et al. 2001; Michna et
2000). Although Ca 1.3 was first cloned in the early 1990s,
al. 2003; Safa et al. 2001; Scholze et al. 2001; Xu and
low expression levels in heterologous systems limited electro-
Lipscombe 2001).
physiological studies of this L-type calcium channel (Hui et al.
1991; Williams et al. 1992). Aside from a report that Ca 1.3
Ca 1.3 L-type channels are only partially inhibited
L-type channels could be reversibly inhibited by the N-type
calcium channel blocker -conotoxin GVIA (Williams et al.
1992), a result that has not been confirmed (Xu and Lipscombe
All L-type calcium channels studied to date are sensitive to
2001), Ca 1.3 channels were not considered unique.
dihydropyridine antagonists and agonists. However, Ca 1.3-
J Neurophysiol • VOL 92 • NOVEMBER 2004 • www.jn.org
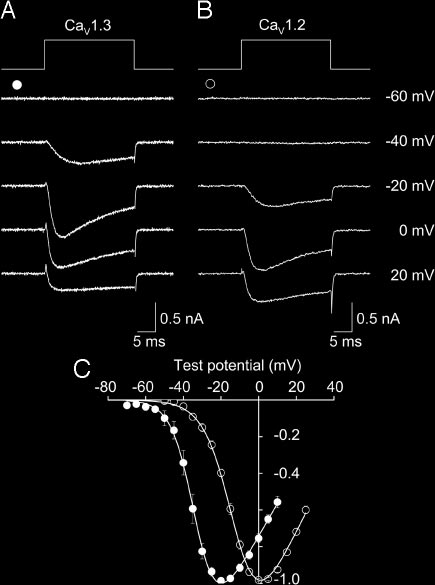
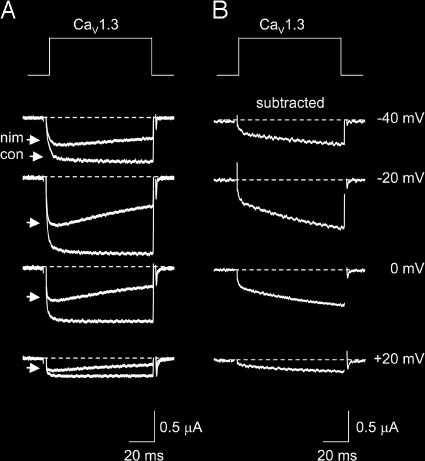
D. LIPSCOMBE, T. D. HELTON, AND W. XU
currents that resemble the R-type current of many neurons(Foehring et al. 2000; Randall and Tsien 1995; Tottene et al.
1996; Yasuda et al. 2003; Zhuravleva et al. 2001).
Ca 1.3 L-type channels open with rapid kinetics
L-type calcium channels in neurons are typically thought of
as slowly activating (Mermelstein et al. 2000; Yasuda et al.
2003). If true, this property limits the involvement of L-typecalcium channels to signaling pathways triggered by moreprolonged membrane depolarizations. However, Ca 1.2 and
Ca 1.3 currents shown in Fig. 2 clearly activate with fast
kinetics (Xu and Lipscombe 2001) and, although contrary tothe pervading viewpoint, these data are consistent with certainother studies. Cloned Ca 1.2 L-type channels have been shown
to support at least as much calcium influx as Ca 2 channels, in
response to brief action potential stimuli (Liu et al. 2003) andnative L-type channels mediate spike-induced calcium influx inhippocampal dendrites (Christie et al. 1995). It is likely thatactivation kinetics of L-type calcium channels will vary de-pending on several factors including cell-type, temperature,alternative splicing, and the presence of auxiliary subunits(Birnbaumer et al. 1998; Lipscombe 2002b; Liu et al. 2003).
For example, the Ca 1.2 clone isolated from rabbit heart used
in our earlier studies (Xu and Lipscombe 2001) activates withkinetics that are slow as compared with our neuronal Ca 1.2
Ca 1.3 and Ca 1.2 L-type channels have different activation thresh-
clone (Fig. 2). However, we also suggest that pharmacological
olds. Whole cell currents measured from tsA201 cells expressing Ca 1.3 (A)
subtraction methods used frequently to isolate L-type calcium
and Ca 1.2 (B) together with Ca ␣ ␦ and Ca  . Currents were activated by
channels from other subtypes of voltage-gated calcium chan-
step depolarizations to the indicated test potentials from a holding potential of
⫺100 mV; 2 mM Ca2⫹ was the charge carrier. C: averaged current–voltage
nels in neurons, might have contributed to the notion that
relationships for Ca 1.3 () and Ca 1.2 (E) channels. Activation V
L-type calcium channels activate with slow kinetics (Fig. 3).
calculated from Boltzmann-linear fits were, ⫺40.4 ⫾ 0.9 mV and ⫺16.1 ⫾ 0.5mV for Ca 1.3 and Ca 1.2, respectively (n ⫽ 8, 11). For a more detailed
description of methods see Xu and Lipscombe (2001). All clones were isolatedin our laboratory. Ca 1.3 and Ca ␣ ␦ clones were isolated from a rat
sympathetic cDNA library, Ca 1.2 from mouse brain, and Ca  from rat
brain. Accession numbers are for Ca 1.3: AF370009; Ca 1.2: AY728090;
Ca  is identical to M88751; Ca ␣ ␦ : AF286488.
containing L-type calcium channels appear to be significantlyless sensitive to dihydropyridine antagonists (Koschak et al.
2001; Xu and Lipscombe 2001). This property complicatesidentification of Ca 1.3 currents. For example, ⬎90% of
Ca 1.2 current is inhibited by 1 M nimodipine, but this same
concentration inhibits only 50% of peak Ca 1.3 current (Xu
and Lipscombe 2001). Striessnig and colleagues obtained sim-ilar results using isradipine (Koschak et al. 2001). The lowersensitivity of Ca 1.3 channels to dihydropyridine antagonists
becomes even more significant at hyperpolarized membranepotentials. Inhibition by dihydropyridines is state-dependent:enhanced at depolarized membrane potentials that open thechannel, but reduced at hyperpolarized membrane potentials(Bean 1984; Berjukow et al. 2000). Consequently, dihydro-pyridines become particularly ineffective at inhibiting Ca 1.3
currents activated at the foot of the current–voltage curve (Xu
Dihydropyridine-sensitive component of the Ca 1.3 L-type current
appears to activate slowly. A: representative Ca 1.3 currents in the absence
and Lipscombe 2001). Interestingly, the Ca 1.3 current that
(black) and presence (gray) of 1 M nifedipine. Currents were activated by
remains in the presence of dihydropyridines takes on the profile
step depolarizations to the indicated potentials from a holding potential of
of an inactivating current with barium as the charge carrier
⫺100 mV. Currents were recorded from Xenopus oocytes expressing Ca 1.3,
(Fig. 3) (Xu and Lipscombe 2001). This is consistent with the
Ca ␣ ␦, and Ca  ; 5 mM barium is the charge carrier. Additional details can
state-dependent nature of the block by dihydropyridines (Bean
be found in Xu and Lipscombe (2001). B: subtracted Ca 1.3 currents showing
the dihydropyridine-sensitive component at the indicated test potentials. Cur-
1984; Berjukow and Hering 2001). In their presence, Ca 1.3
rents appear to activate slowing because of the time-dependent nature of
channels generate low-threshold, drug-resistant, inactivating
dihydropyridine block. Scale bars: 0.5 A, 20 ms.
J Neurophysiol • VOL 92 • NOVEMBER 2004 • www.jn.org
L-TYPE CALCIUM CHANNELS
The L-type calcium current in neurons is defined frequently as
Avery and Johnston commented that ". . the designation ‘low-
the whole cell calcium current that is inhibited by dihydropyr-
voltage-activated' should not be limited to T-type channels."
idine antagonists. Pharmacological subtraction is only appro-
These investigators ". . challenge the traditional designation
priate, however, when inhibition is complete, and independent
of L-type channels as exclusively HVA and reveal a possible
of voltage and time; conditions not met for dihydropyridine
role in subthreshold Ca2⫹ signaling" (Avery and Johnston 1996).
block of Ca 1.3 L-type currents (Figs. 2, 3). Figure 3 illustrates
Single-channel recordings also indicate differences among
that Ca 1.3 L-type currents open with rapid kinetics, and
neuronal L-type calcium channels in hippocampal pyramidal
inactivate little with barium as the charge carrier. However, in
and cerebellar granule cells (Forti and Pietrobon 1993; Kava-
the presence of nimodipine, inhibition is incomplete and the
lali and Plummer 1996; Schjott and Plummer 2000). Record-
remaining current appears to inactivate. These data are consis-
ings distinguish at least 2 gating activities of L-type calcium
tent with the state-dependent nature of nimodipine block.
channels that may represent different channel subtypes. It will
Consequently, the dihydropyridine-sensitive, subtracted cur-
be interesting to determine their molecular origins.
rent appears as slowly activating, not because L-type calciumchannels open slowly but because the block is time-dependent
and incomplete (Fig. 3).
Neuronal L-type calcium channels play established roles in
Ca 1.4 L-type channels are functionally unique
regulating gene expression, cell survival, and synaptic plastic-
ity (Christie et al. 1997; Deisseroth et al. 1998; Galli et al.
Ca 1.4 was recently cloned from human and mouse retinal
1995; Mao et al. 1999; Marshall et al. 2003; Murphy et al.
tissue and heterologously expressed in mammalian cells (Bau-
1991; Norris et al. 1998; Weisskopf et al. 1999). In select cells
mann et al. 2004; Koschak et al. 2003; McRory et al. 2004).
and synapses, L-type calcium channels can also regulate exo-
Although currents generated from cells expressing Ca 1.4
cytosis (Ashcroft et al. 1994; Fuchs 1996; Heidelberger and
clones were small, they had properties similar in several
Matthews 1992; Liu et al. 2004; Sand et al. 2001; Thaler et al.
respects to those of Ca 1.3. These include rapid activation
2001; Wiser et al. 1999). In addition, data reviewed here
kinetics, low activation threshold, and lower sensitivity to
suggest Ca 1.3 L-type calcium channels mediate subthreshold
dihydropyridine inhibition (Baumann et al. 2004; Koschak et
calcium signaling. For example, dihydropyridine antagonists
al. 2003; McRory et al. 2004). Native L-type currents in retinal
suppress spontaneous intracellular calcium oscillations and
cells, presumed to be Ca 1.4-containing (Taylor and Morgans
slow rhythmic firing in several excitable cells, including cere-
1998; Wilkinson and Barnes 1996), are likewise similar to
bellar Purkinje neurons, suprachiasmatic nucleus neurons, in-
recombinant Ca 1.3 and Ca 1.4 channels. They have lower
ferior olivary neurons, corticostriatial neurons, pituitary cells,
sensitivity to dihydropyridines and activate at negative thresh-
and GH3 cells (Charles et al. 1999; Giraldez et al. 2002;
olds. These data are consistent with the relatively high level of
Liljelund et al. 2000; Pennartz et al. 2002; Placantonakis and
sequence homology between Ca 1.3 and Ca 1.4 genes (Fig. 1)
Welsh 2001; Vergara et al. 2003). These studies and those that
(Lipscombe 2002b). Heterologously expressed Ca 1.4 L-type
show sinoatrial node dysfunction in Ca 1.3 knockout mice
calcium channels are also distinctive in lacking calcium-de-
(Mangoni et al. 2003; Platzer et al. 2000; Zhang et al. 2002)
pendent inactivation (Baumann et al. 2004; Koschak et al.
strongly implicate Ca 1.3 L-type calcium channels in driving
2003; McRory et al. 2004). The currents in these studies were
oscillatory activity. Ca 1.3 channels could also mediate sus-
all relatively small but calcium entering through a single
tained calcium entry during action potential plateaus, as calci-
channel should be sufficient to support inactivation, if compo-
um-dependent and voltage-dependent inactivation is minimal
nents of the calcium-dependent inactivation pathway are
at depolarized voltages (Figs. 2 and 3).
present (Peterson et al. 2000; Yue et al. 1990). The absence of
Many neurons express low- to mid-threshold activating,
calcium-dependent inactivation suggests that Ca 1.4 channels
inactivating, and drug-resistant calcium currents collectively
are functionally distinct from Ca 1.3 and Ca 1.2 channels
called R-type. These currents are generally attributed to Ca 2.3
(Koschak et al. 2003; McRory et al. 2004).
channels (Cloues and Sather 2003; Magistretti et al. 2000;Randall and Tsien 1995; Tottene et al. 1996; Yasuda et al.
Native neuronal L-type currents are functionally diverse
2003). The properties of Ca 1.3 channels suggest that distin-
guishing them from Ca 2.3 channels using dihydropyridine
Certain cell-types, including hair cells, amacrine cells, and
antagonists would be difficult. It is quite likely that Ca 1.3
endocrine cells express a limited number of calcium channels.
L-type calcium channels contribute a significant fraction of the
Within this background, low-threshold dihydropyridine-sensi-
R-type current in many neurons. The prevalence of significant
tive L-type calcium currents are more readily identified (Ash-
drug-resistant R-type currents in neurons of Ca 2.3 knockout
croft et al. 1994; Habermann et al. 2003; Liu et al. 2004;
mice strongly supports this proposal (Wilson et al. 2000).
Michna et al. 2003; Platzer et al. 2000; Schnee and Ricci 2003;
R-type currents contribute to presynaptic transmitter release at
Smith et al. 1993). Several groups also report low-threshold-
certain synapses (Wu et al. 1999) and to synaptic plasticity in
activating dihydropyridine-sensitive L-type currents in hip-
dendritic spines of hippocampal pyramidal neurons (Yasuda et
pocampal pyramidal, cortical striatal, suprachiasmatic, tha-
lamic, and motor neurons with properties similar to Ca 1.3
Ca 1.3 L-type channels will activate in response to physi-
channels (Avery and Johnston 1996; Cloues and Sather 2003;
ological stimuli that do not open Ca 1.2 L-type channels. This
Li and Bennett 2003; Liljelund et al. 2000; Pennartz et al.
broadens the functional importance of L-type calcium channels
2002; Sand et al. 2001; Svirskis and Hounsgaard 1997; Ver-
to included neuronal processes triggered by fast, subthreshold
gara et al. 2003; Zhuravleva et al. 2001). Notably in 1996,
depolarizations. Differences in their primary structure and
J Neurophysiol • VOL 92 • NOVEMBER 2004 • www.jn.org
D. LIPSCOMBE, T. D. HELTON, AND W. XU
subcellular distributions also indicate that Ca 1.2 and Ca 1.3
Berntson A, Taylor WR, and Morgans CW. Molecular identity, synaptic
channels are likely to regulate divergent downstream signaling
localization, and physiology of calcium channels in retinal bipolar cells.
pathways. It will be very interesting to know, for example, if
J Neurosci Res 71: 146 –151, 2003.
Biel M, Ruth P, Bosse E, Hullin R, Stuhmer W, Flockerzi V, and Hofmann
Ca 1.3 channels can couple to transcription factors as effi-
F. Primary structure and functional expression of a high voltage activated
ciently as Ca 1.2 (Dolmetsch et al. 2001; Weick et al. 2003).
calcium channel from rabbit lung. FEBS Lett 269: 409 – 412, 1990.
While we wait for the low down on Ca 1.3 channels, a
Birnbaumer L, Qin N, Olcese R, Tareilus E, Platano D, Costantin J, and
selective inhibitor to differentiate between L-type calcium
Stefani E. Structures and functions of calcium channel beta subunits.
channel subtypes would be invaluable for establishing their
J Bioenerg Biomembr 30: 357–375, 1998.
relative contributions to calcium signaling in neurons.
Bourinet E, Charnet P, Tomlinson WJ, Stea A, Snutch TP, and Nargeot J.
Voltage-dependent facilitation of a neuronal alpha 1C L-type calcium
channel. EMBO J 13: 5032–5039, 1994.
Bowden SEH, Fletcher S, Loane DJ, and Marrion NV. Somatic colocal-
We are grateful to members of the Lipscombe laboratory for constructive
ization of rat SK1 and D class (Cav 1.2) L-type calcium channels in rat CA1
criticisms and particularly to A. Gray for helping generate the Ca 1 gene tree.
hippocampal pyramidal neurons. J Neurosci 21: 175RC, 2001.
A. Castiglioni and A. Amenta obtained the Ca 1.2 clone used in these studies.
Charles AC, Piros ET, Evans CJ, and Hales TG. L-type Ca2⫹ channels and
Present address of W. Xu: Nancy Friend Pritzker Laboratory, Department of
K⫹ channels specifically modulate the frequency and amplitude of sponta-
Psychiatry and Behavioral Sciences, Stanford University School of Medicine,
neous Ca2⫹ oscillations and have distinct roles in prolactin release in GH3
Palo Alto, CA 94304-5485.
cells. J Biol Chem 274: 7508 –7515, 1999.
Charnet P, Bourinet E, Dubel SJ, Snutch TP, and Nargeot J. Calcium
currents recorded from a neuronal alpha 1C L-type calcium channel in
This work was supported by National Institute of Neurological Disorders
Xenopus oocytes. FEBS Lett 344: 87–90, 1994.
and Stroke Grant NS-29967.
Christie BR, Eliot LS, Ito K, Miyakawa H, and Johnston D. Different Ca2⫹
channels in soma and dendrites of hippocampal pyramidal neurons mediate
spike-induced Ca2⫹ influx. J Neurophysiol 73: 2553–2557, 1995.
Adams BA, Tanabe T, Mikami A, Numa S, and Beam KG. Intramembrane
Christie BR, Schexnayder LK, and Johnston D. Contribution of voltage-
charge movement restored in dysgenic skeletal muscle by injection of
gated Ca2⫹ channels to homosynaptic long-term depression in the CA1
dihydropyridine receptor cDNAs. Nature 346: 569 –572, 1990.
region in vitro. J Neurophysiol 77: 1651–1655, 1997.
Almers W and Palade PT. Slow calcium and potassium currents across frog
Cloues RK and Sather WA. Afterhyperpolarization regulates firing rate in
muscle membrane: measurements with a Vaseline-gap technique. J Physiol
neurons of the suprachiasmatic nucleus. J Neurosci 23: 1593–1604, 2003.
312: 159 –176, 1981.
Cruz LJ, Johnson DS, and Olivera BM. Characterization of the omega-
Altier C, Spaetgens RL, Nargeot J, Bourinet E, and Zamponi GW.
conotoxin target. Evidence for tissue-specific heterogeneity in calcium
Multiple structural elements contribute to voltage-dependent facilitation of
channel types. Biochemistry 26: 820 – 824, 1987.
neuronal alpha 1C (CaV1.2) L-type calcium channels. Neuropharmacology
De Koninck P, and Cooper E. Differential regulation of neuronal nicotinic
40: 1050 –1057, 2001.
ACh receptor subunit genes in cultured neonatal rat sympathetic neurons:
Ashcroft FM, Proks P, Smith PA, Ammala C, Bokvist K, and Rorsman P.
specific induction of alpha 7 by membrane depolarization through a Ca2⫹/
Stimulus-secretion coupling in pancreatic beta cells. J Cell Biochem 55:
calmodulin-dependent kinase pathway. J Neurosci 15: 7966 –7978, 1995.
54 – 65, 1994.
de Leon M, Wang Y, Jones L, Perez-Reyes E, Wei X, Soong TW, Snutch
Avery RB and Johnston D. Multiple channel types contribute to the low-
TP, and Yue DT. Essential Ca(2⫹)-binding motif for Ca(2⫹)-sensitive
voltage-activated calcium current in hippocampal CA3 pyramidal neurons.
inactivation of L-type Ca2⫹ channels. Science 270: 1502–1506, 1995.
J Neurosci 16: 5567–5582, 1996.
Deisseroth K, Heist EK, and Tsien RW. Translocation of calmodulin to the
Bading H, Ginty DD, and Greenberg ME. Regulation of gene expression in
nucleus supports CREB phosphorylation in hippocampal neurons. Nature
hippocampal neurons by distinct calcium signaling pathways. Science 260:
392: 198 –202, 1998.
181–186, 1993.
Diebold RJ, Koch WJ, Ellinor PT, Wang JJ, Muthuchamy M, Wieczorek
Baumann L, Gerstner A, Zong X, Biel M, and Wahl-Schott C. Functional
DF, and Schwartz A. Mutually exclusive exon splicing of the cardiac
characterization of the L-type Ca2⫹ channel Cav1.4alpha1 from mouse
calcium channel alpha 1 subunit gene generates developmentally regulated
retina. Invest Ophthalmol Vis Sci 45: 708 –713, 2004.
isoforms in the rat heart. Proc Natl Acad Sci USA 89: 1497–1501, 1992.
Beam KG, Tanabe T, and Numa S. Structure, function, and regulation of the
Dolmetsch RE, Pajvani U, Fife K, Spotts JM, and Greenberg ME.
skeletal muscle dihydropyridine receptor. Ann NY Acad Sci 560: 127–137,
Signaling to the nucleus by an L-type calcium channel-calmodulin complex
through the MAP kinase pathway. Science 294: 333–339, 2001.
Bean BP. Nitrendipine block of cardiac calcium channels: high-affinity bind-
Dunlap K, Luebke JI, and Turner TJ. Exocytotic Ca2⫹ channels in mam-
ing to the inactivated state. Proc Natl Acad Sci USA 81: 6388 – 6392, 1984.
malian central neurons. Trends Neurosci 18: 89 –98, 1995.
Bean BP. Classes of calcium channels in vertebrate cells. Annu Rev Physiol
Ertel EA, Campbell KP, Harpold MM, Hofmann F, Mori Y, Perez-Reyes
51: 367–384, 1989.
E, Schwartz A, Snutch TP, Tanabe T, Birnbaumer L, Tsien RW, and
Bean BP. Pharmacology of calcium channels in cardiac muscle, vascular
Catterall WA. Nomenclature of voltage-gated calcium channels. Neuron
muscle, and neurons. Am J Hypertens 4: 406S– 411S, 1991.
25: 533–535, 2000.
Bech-Hansen NT, Naylor MJ, Maybaum TA, Pearce WG, Koop B,
Finkbeiner S and Greenberg ME. Ca2⫹ channel-regulated neuronal gene
Fishman GA, Mets M, Musarella MA, and Boycott KM. Loss-of-
expression. J Neurobiol 37: 171–189, 1998.
function mutations in a calcium-channel alpha1-subunit gene in Xp11.23
Flucher BE and Franzini-Armstrong C. Formation of junctions involved in
cause incomplete X-linked congenital stationary night blindness. Nat Genet
excitation-contraction coupling in skeletal and cardiac muscle. Proc Natl
19: 264 –267, 1998.
Acad Sci USA 93: 8101– 8106, 1996.
Bell DC, Butcher AJ, Berrow NS, Page KM, Brust PF, Nesterova A,
Foehring RC, Mermelstein PG, Song WJ, Ulrich S, and Surmeier DJ.
Stauderman KA, Seabrook GR, Nurnberg B, and Dolphin AC. Biophys-
Unique properties of R-type calcium currents in neocortical and neostriatal
ical properties, pharmacology, and modulation of human, neuronal L-type
neurons. J Neurophysiol 84: 2225–2236, 2000.
(alpha(1D), Ca(V)1.3) voltage-dependent calcium currents. J Neurophysiol
Forti L and Pietrobon D. Functional diversity of L-type calcium channels in
85: 816 – 827, 2001.
rat cerebellar neurons. Neuron 10: 437– 450, 1993.
Berjukow S and Hering S. Voltage-dependent acceleration of Ca(v)1.2
Frankenhaeuser B and Hodgkin AL. The action of calcium on the electrical
channel current decay by (⫹)- and (⫺)-isradipine. Br J Pharmacol 133:
properties of squid axons. J Physiol 137: 218 –244, 1957.
959 –966, 2001.
Franzini-Armstrong C and Protasi F. Ryanodine receptors of striated
Berjukow S, Marksteiner R, Gapp F, Sinnegger MJ, and Hering S.
muscles: a complex channel capable of multiple interactions. Physiol Rev
Molecular mechanism of calcium channel block by isradipine. Role of a
77: 699 –729, 1997.
drug-induced inactivated channel conformation. J Biol Chem 275: 22114 –
Fuchs PA. Synaptic transmission at vertebrate hair cells. Curr Opin Neurobiol
22120, 2000.
6: 514 –519, 1996.
J Neurophysiol • VOL 92 • NOVEMBER 2004 • www.jn.org
L-TYPE CALCIUM CHANNELS
Galli C, Meucci O, Scorziello A, Werge TM, Calissano P, and Schettini G.
Liu Z, Ren J, and Murphy TH. Decoding of synaptic voltage waveforms by
Apoptosis in cerebellar granule cells is blocked by high KCl, forskolin, and
specific classes of recombinant high-threshold Ca(2⫹) channels. J Physiol
IGF-1 through distinct mechanisms of action: the involvement of intracel-
553: 473– 488, 2003.
lular calcium and RNA synthesis. J Neurosci 15: 1172–1179, 1995.
Ludwig A, Flockerzi V, and Hofmann F. Regional expression and cellular
Giraldez T, de la Pena P, Gomez-Varela D, and Barros F. Correlation
localization of the alpha1 and beta subunit of high voltage-activated calcium
between electrical activity and intracellular Ca2⫹ oscillations in GH3 rat
channels in rat brain. J Neurosci 17: 1339 –1349, 1997.
anterior pituitary cells. Cell Calcium 31: 65–78, 2002.
Magistretti J, Brevi S, and de Curtis M. A blocker-resistant, fast-decaying,
Habermann CJ, O'Brien BJ, Wassle H, and Protti DA. AII amacrine cells
intermediate-threshold calcium current in palaeocortical pyramidal neurons.
express L-type calcium channels at their output synapses. J Neurosci 23:
Eur J Neurosci 12: 2376 –2386, 2000.
6904 – 6913, 2003.
Mangoni ME, Couette B, Bourinet E, Platzer J, Reimer D, Striessnig J,
Heidelberger R and Matthews G. Calcium influx and calcium current in
and Nargeot J. Functional role of L-type Cav1.3 Ca2⫹ channels in cardiac
single synaptic terminals of goldfish retinal bipolar neurons. J Physiol 447:
pacemaker activity. Proc Natl Acad Sci USA 100: 5543–5548, 2003.
235–256, 1992.
Mao Z, Bonni A, Xia F, Nadal-Vicens M, and Greenberg ME. Neuronal
Hell JW, Westenbroek RE, Warner C, Ahlijanian MK, Prystay W,
activity-dependent cell survival mediated by transcription factor MEF2.
Gilbert MM, Snutch TP, and Catterall WA. Identification and differential
Science 286: 785–790, 1999.
subcellular localization of the neuronal class C and class D L-type calcium
Marrion NV and Tavalin SJ. Selective activation of Ca2⫹-activated K⫹
channel alpha 1 subunits. J Cell Biol 123: 949 –962, 1993.
channels by co-localized Ca2⫹ channels in hippocampal neurons. Nature
Hille B. Ion Channels of Excitable Membranes. Sunderland, MA: Sinauer,
395: 900 –905, 1998.
Marshall J, Dolan BM, Garcia EP, Sathe S, Tang X, Mao Z, and Blair LA.
Hui A, Ellinor PT, Krizanova O, Wang JJ, Diebold RJ, and Schwartz A.
Calcium channel and NMDA receptor activities differentially regulate
Molecular cloning of multiple subtypes of a novel rat brain isoform of the
nuclear C/EBPbeta levels to control neuronal survival. Neuron 39: 625– 639,
alpha 1 subunit of the voltage-dependent calcium channel. Neuron 7: 35– 44,
Mathur R, Zheng J, Yan Y, and Sigworth FJ. Role of the S3–S4 linker in
Ihara Y, Yamada Y, Fujii Y, Gonoi T, Yano H, Yasuda K, Inagaki N,
Shaker potassium channel activation. J Gen Physiol 109: 191–199, 1997.
Seino Y, and Seino S. Molecular diversity and functional characterization
McIntosh JM, Olivera BM, and Cruz LJ. Conus peptides as probes for ion
of voltage-dependent calcium channels (CACN4) expressed in pancreatic
channels. Methods Enzymol 294: 605– 624, 1999.
beta-cells. Mol Endocrinol 9: 121–130, 1995.
McRory JE, Hamid J, Doering CJ, Garcia E, Parker R, Hamming K,
Ivanina T, Blumenstein Y, Shistik E, Barzilai R, and Dascal N. Modulation
Chen L, Hildebrand M, Beedle AM, Feldcamp L, Zamponi GW, and
of L-type Ca2⫹ channels by gbeta gamma and calmodulin via interactions
Snutch TP. The CACNA1F gene encodes an L-type calcium channel with
with N and C termini of alpha 1C. J Biol Chem 275: 39846 –39854, 2000.
unique biophysical properties and tissue distribution. J Neurosci 24: 1707–
Kamsler A and Segal M. Hydrogen peroxide modulation of synaptic plastic-
ity. J Neurosci 23: 269 –276, 2003.
Mermelstein PG, Bito H, Deisseroth K, and Tsien RW. Critical dependence
Kanngiesser U, Nalik P, and Pongs O. Purification and affinity labeling of
of cAMP response element-binding protein phosphorylation on L-type
dihydropyridine receptor from rabbit skeletal muscle membranes. Proc Natl
calcium channels supports a selective response to EPSPs in preference to
Acad Sci USA 85: 2969 –2973, 1988.
action potentials. J Neurosci 20: 266 –273, 2000.
Kavalali ET and Plummer MR. Multiple voltage-dependent mechanisms
Michna M, Knirsch M, Hoda JC, Muenkner S, Langer P, Platzer J,
potentiate calcium channel activity in hippocampal neurons. J Neurosci 16:
Striessnig J, and Engel J. Cav1.3 (alpha1D) Ca2⫹ currents in neonatal
1072–1082, 1996.
outer hair cells of mice. J Physiol 553: 747–758, 2003.
Koch WJ, Ellinor PT, and Schwartz A. cDNA cloning of a dihydropyridine-
Mikami A, Imoto K, Tanabe T, Niidome T, Mori Y, Takeshima H,
sensitive calcium channel from rat aorta. Evidence for the existence of
Narumiya S, and Numa S. Primary structure and functional expression of
alternatively spliced forms. J Biol Chem 265: 17786 –17791, 1990.
the cardiac dihydropyridine-sensitive calcium channel. Nature 340: 230 –
Kollmar R, Fak J, Montgomery LG, and Hudspeth AJ. Hair cell-specific
splicing of mRNA for the alpha1D subunit of voltage-gated Ca2⫹ channels
Mintz IM, Venema VJ, Swiderek KM, Lee TD, Bean BP, and Adams ME.
in the chicken's cochlea. Proc Natl Acad Sci USA 94: 14889 –14893, 1997a.
P-type calcium channels blocked by the spider toxin omega-Aga-IVA.
Kollmar R, Montgomery LG, Fak J, Henry LJ, and Hudspeth AJ.
Nature 355: 827– 829, 1992.
Predominance of the alpha1D subunit in L-type voltage-gated Ca2⫹ chan-
Morgans CW. Calcium channel heterogeneity among cone photoreceptors in
nels of hair cells in the chicken's cochlea. Proc Natl Acad Sci USA 94:
the tree shrew retina. Eur J Neurosci 11: 2989 –2993, 1999.
Morgans CW, El Far O, Berntson A, Wassle H, and Taylor WR. Calcium
Koschak A, Reimer D, Huber I, Grabner M, Glossmann H, Engel J, and
extrusion from mammalian photoreceptor terminals. J Neurosci 18: 2467–
Striessnig J. Alpha 1D (Cav1.3) subunits can form l-type Ca2⫹ channels
activating at negative voltages. J Biol Chem 276: 22100 –22106, 2001.
Mori Y, Niidome T, Fujita Y, Mynlieff M, Dirksen RT, Beam KG, Iwabe
Koschak A, Reimer D, Walter D, Hoda JC, Heinzle T, Grabner M, and
N, Miyata T, Furutama D, Furuichi T, and Mikoshiba K. Molecular
Striessnig J. Cav1.4alpha1 subunits can form slowly inactivating dihydro-
diversity of voltage-dependent calcium channel. Ann NY Acad Sci 707:
pyridine-sensitive L-type Ca2⫹ channels lacking Ca2⫹-dependent inactiva-
87–108, 1993.
tion. J Neurosci 23: 6041– 6049, 2003.
Murakami M, Nakagawasai O, Fujii S, Kameyama K, Murakami S,
Lei S, Pelkey KA, Topolnik L, Congar P, Lacaille JC, and McBain CJ.
Hozumi S, Esashi A, Taniguchi R, Yanagisawa T, Tan-no K, Tadano T,
Depolarization-induced long-term depression at hippocampal mossy fiber-
Kitamura K, and Kisara K. Antinociceptive action of amlodipine blocking
CA3 pyramidal neuron synapses. J Neurosci 23: 9786 –9795, 2003.
N-type Ca2⫹ channels at the primary afferent neurons in mice. Eur J Phar-
Li Y and Bennett DJ. Persistent sodium and calcium currents cause plateau
macol 419: 175–181, 2001.
potentials in motoneurons of chronic spinal rats. J Neurophysiol 90: 857–
Murphy TH, Worley PF, and Baraban JM. L-type voltage-sensitive calcium
channels mediate synaptic activation of immediate early genes. Neuron 7:
Liljelund P, Netzeband JG, and Gruol DL. L-type calcium channels mediate
625– 635, 1991.
calcium oscillations in early postnatal Purkinje neurons. J Neurosci 20:
Nakai J, Adams BA, Imoto K, and Beam KG. Critical roles of the S3
7394 –7403, 2000.
segment and S3–S4 linker of repeat I in activation of L-type calcium
Lipscombe D. L-type calcium channels: highs and new lows. Circ Res 90:
channels. Proc Natl Acad Sci USA 91: 1014 –1018, 1994.
933–935, 2002a.
Norris CM, Halpain S, and Foster TC. Reversal of age-related alterations in
Lipscombe D, Pan QJ, and Gray AC. Functional diversity in neuronal
synaptic plasticity by blockade of L-type Ca2⫹ channels. J Neurosci 18:
voltage-gated calcium channels by alternative splicing of Cav alpah1. Mol
3171–3179, 1998.
Neurobiol. 26: 21– 44, 2002b.
Ouardouz M, Nikolaeva MA, Coderre E, Zamponi GW, McRory JE,
Liu G, Hilliard N, and Hockerman GH. Cav1.3 is preferentially coupled to
Trapp BD, Yin X, Wang W, Woulfe J, and Stys PK. Depolarization-
glucose-induced [Ca2⫹] oscillations in the pancreatic  cell line INS-1. Mol
induced Ca2⫹ release in ischemic spinal cord white matter involves L-type
Pharmacol 65: 1269 –1277, 2004.
Ca2⫹ channel activation of ryanodine receptors. Neuron 40: 53– 63, 2003.
J Neurophysiol • VOL 92 • NOVEMBER 2004 • www.jn.org
D. LIPSCOMBE, T. D. HELTON, AND W. XU
Pennartz CM, de Jeu MT, Bos NP, Schaap J, and Geurtsen AM. Diurnal
Zrenner E, Meitinger T, Rosenthal A, and Meindl A. An L-type calcium-
modulation of pacemaker potentials and calcium current in the mammalian
channel gene mutated in incomplete X-linked congenital stationary night
circadian clock. Nature 416: 286 –290, 2002.
blindness. Nat Genet 19: 260 –263, 1998.
Perez-Reyes E, Cribbs LL, Daud A, Lacerda AE, Barclay J, Williamson
Svirskis G and Hounsgaard J. Depolarization-induced facilitation of a
MP, Fox M, Rees M, and Lee JH. Molecular characterization of a neuronal
plateau-generating current in ventral horn neurons in the turtle spinal cord.
low-voltage-activated T-type calcium channel. Nature 391: 896 –900, 1998.
J Neurophysiol 78: 1740 –1742, 1997.
Perez-Reyes E, Wei XY, Castellano A, and Birnbaumer L. Molecular
Tachibana M, Okada T, Arimura T, and Kobayashi K. Dihydropyridine-
diversity of L-type calcium channels. Evidence for alternative splicing of the
sensitive calcium current mediates neurotransmitter release from retinal
transcripts of three non-allelic genes. J Biol Chem 265: 20430 –20436, 1990.
bipolar cells. Ann NY Acad Sci 707: 359 –361, 1993.
Peterson BZ, Lee JS, Mulle JG, Wang Y, de Leon M, and Yue DT. Critical
Takimoto K, Li D, Nerbonne JM, and Levitan ES. Distribution, splicing and
determinants of Ca(2⫹)-dependent inactivation within an EF-hand motif of
glucocorticoid-induced expression of cardiac alpha 1C and alpha 1D volt-
L-type Ca(2⫹) channels. Biophys J 78: 1906 –1920, 2000.
age-gated Ca2⫹ channel mRNAs. J Mol Cell Cardiol 29: 3035–3042, 1997.
Placantonakis D and Welsh J. Two distinct oscillatory states determined by
Tanabe T, Beam KG, Powell JA, and Numa S. Restoration of excitation-
the NMDA receptor in rat inferior olive. J Physiol 534: 123–140, 2001.
contraction coupling and slow calcium current in dysgenic muscle by
Platzer J, Engel J, Schrott-Fischer A, Stephan K, Bova S, Chen H, Zheng
dihydropyridine receptor complementary DNA. Nature 336: 134 –139,
H, and Striessnig J. Congenital deafness and sinoatrial node dysfunction in
mice lacking class D L-type Ca2⫹ channels. Cell 102: 89 –97, 2000.
Tanabe T, Mikami A, Numa S, and Beam KG. Cardiac-type excitation-
Randall A and Tsien RW. Pharmacological dissection of multiple types of
contraction coupling in dysgenic skeletal muscle injected with cardiac
Ca2⫹ channel currents in rat cerebellar granule neurons. J Neurosci 15:
dihydropyridine receptor cDNA. Nature 344: 451– 453, 1990.
2995–3012, 1995.
Tanabe T, Takeshima H, Mikami A, Flockerzi V, Takahashi H, Kangawa
Reuter H. A variety of calcium channels. Nature 316: 391, 1985.
Reuter H, Porzig H, Kokubun S, and Prod'hom B. Calcium channels in the
K, Kojima M, Matsuo H, Hirose T, and Numa S. Primary structure of the
heart. Properties and modulation by dihydropyridine enantiomers. Ann NY
receptor for calcium channel blockers from skeletal muscle. Nature 328:
Acad Sci 522: 16 –24, 1988.
313–318, 1987.
Rios E and Brum G. Involvement of dihydropyridine receptors in excitation-
Tang CY and Papazian DM. Transfer of voltage independence from a rat
contraction coupling in skeletal muscle. Nature 325: 717–720, 1987.
olfactory channel to the Drosophila ether-a-go-go K⫹ channel. J Gen
Russo G, Lelli A, Gioglio L, and Prigioni I. Nature and expression of
Physiol 109: 301–311, 1997.
dihydropyridine-sensitive and -insensitive calcium currents in hair cells of
Taylor WR and Morgans C. Localization and properties of voltage-gated
frog semicircular canals. Pfluegers Arch 446: 189 –197, 2003.
calcium channels in cone photoreceptors of Tupaia belangeri. Vis Neurosci
Safa P, Boulter J, and Hales TG. Functional properties of Cav1.3 (alpha1D)
15: 541–552, 1998.
L-type Ca2⫹ channel splice variants expressed by rat brain and neuroendo-
Thaler C, Li W, and Brehm P. Calcium channel isoforms underlying
crine GH3 cells. J Biol Chem 276: 38727–38737, 2001.
synaptic transmission at embryonic Xenopus neuromuscular junctions.
Sand O, Chen BM, and Grinnell AD. Contribution of L-type Ca(2⫹)
J Neurosci 21: 412– 422, 2001.
channels to evoked transmitter release in cultured Xenopus nerve-muscle
Thibault O, Hadley R, and Landfield PW. Elevated postsynaptic [Ca2⫹] and
synapses. J Physiol 536: 21–33, 2001.
L-type calcium channel activity in aged hippocampal neurons: relationship
Schjott JM and Plummer MR. Sustained activation of hippocampal Lp-type
to impaired synaptic plasticity. J Neurosci 21: 9744 –9756, 2001.
voltage-gated calcium channels by tetanic stimulation. J Neurosci 20:
Tottene A, Moretti A, and Pietrobon D. Functional diversity of P-type and
4786 – 4797, 2000.
R-type calcium channels in rat cerebellar neurons. J Neurosci 16: 6353–
Schnee ME and Ricci AJ. Biophysical and pharmacological characterization
of voltage-gated calcium currents in turtle auditory hair cells. J Physiol 549:697–717, 2003.
Vergara R, Rick C, Hernandez-Lopez S, Laville JA, Guzman JN, Galar-
Schneider MF and Chandler WK. Voltage dependent charge movement of
raga E, Surmeier DJ, and Bargas J. Spontaneous voltage oscillations in
skeletal muscle: a possible step in excitation-contraction coupling. Nature
striatal projection neurons in a rat corticostriatal slice. J Physiol 553:
242: 244 –246, 1973.
169 –182, 2003.
Scholze A, Plant TD, Dolphin AC, and Nurnberg B. Functional expression
Weick JP, Groth RD, Isaksen AL, and Mermelstein PG. Interactions with
and characterization of a voltage-gated CaV1.3 (alpha1D) calcium channel
PDZ proteins are required for L-type calcium channels to activate cAMP
subunit from an insulin-secreting cell line. Mol Endocrinol 15: 1211–1221,
response element-binding protein-dependent gene expression. J Neurosci
23: 3446 –3456, 2003.
Schorge S, Gupta S, Lin Z, McEnery MW, and Lipscombe D. Calcium
Weisskopf MG, Bauer EP, and LeDoux JE. L-type voltage-gated calcium
channel activation stabilizes a neuronal calcium channel mRNA. Nat Neu-
channels mediate NMDA-independent associative long-term potentiation at
rosci 2: 785–790, 1999.
thalamic input synapses to the amygdala. J Neurosci 19: 10512–10519,
Schultz D, Mikala G, Yatani A, Engle DB, Iles DE, Segers B, Sinke RJ,
Weghuis DO, Klockner U, Wakamori M, Wang J-J, Melvin D, Varadi
Welling A, Ludwig A, Zimmer S, Klugbauer N, Flockerzi V, and Hofmann
G, and Schwartz A. Cloning, chromosomal localization, and functional
F. Alternatively spliced IS6 segments of the alpha 1C gene determine the
expression of the alpha 1 subunit of the L-type voltage-dependent calcium
tissue-specific dihydropyridine sensitivity of cardiac and vascular smooth
channel from normal human heart. Proc Natl Acad Sci USA 90: 6228 – 6232,
muscle L-type Ca2⫹ channels. Circ Res 81: 526 –532, 1997.
Westenbroek RE, Hoskins L, and Catterall WA. Localization of Ca2⫹
Schwartz LM, McCleskey EW, and Almers W. Dihydropyridine receptors
channel subtypes on rat spinal motor neurons, interneurons, and nerve
in muscle are voltage-dependent but most are not functional calcium
terminals. J Neurosci 18: 6319 – 6330, 1998.
channels. Nature 314: 747–751, 1985.
Wilkinson MF and Barnes S. The dihydropyridine-sensitive calcium channel
Seino S, Chen L, Seino M, Blondel O, Takeda J, Johnson JH, and Bell GI.
subtype in cone photoreceptors. J Gen Physiol 107: 621– 630, 1996.
Cloning of the alpha 1 subunit of a voltage-dependent calcium channel
Williams ME, Feldman DH, McCue AF, Brenner R, Velicelebi G, Ellis SB,
expressed in pancreatic beta cells. Proc Natl Acad Sci USA 89: 584 –588,
and Harpold MM. Structure and functional expression of alpha 1, alpha 2,
and beta subunits of a novel human neuronal calcium channel subtype.
Shinnick-Gallagher P, McKernan MG, Xie J, and Zinebi F. L-type voltage-
Neuron 8: 71– 84, 1992.
gated calcium channels are involved in the in vivo and in vitro expression of
Wilson SM, Toth PT, Oh SB, Gillard SE, Volsen S, Ren D, Philipson LH,
fear conditioning. Ann NY Acad Sci 985: 135–149, 2003.
Lee EC, Fletcher CF, Tessarollo L, Copeland NG, Jenkins NA, and
Smith PA, Aschroft FM, and Fewtrell CM. Permeation and gating properties
Miller RJ. The status of voltage-dependent calcium channels in alpha 1E
of the L-type calcium channel in mouse pancreatic beta cells. J Gen Physiol
knock-out mice. J Neurosci 20: 8566 – 8571, 2000.
101: 767–797, 1993.
Wiser O, Trus M, Hernandez A, Renstrom E, Barg S, Rorsman P, and
Soldatov NM. Molecular diversity of L-type Ca2⫹ channel transcripts in
Atlas D. The voltage sensitive Lc-type Ca2⫹ channel is functionally coupled
human fibroblasts. Proc Natl Acad Sci USA 89: 4628 – 4632, 1992.
to the exocytotic machinery. Proc Natl Acad Sci USA 96: 248 –253, 1999.
Strom TM, Nyakatura G, Apfelstedt-Sylla E, Hellebrand H, Lorenz B,
Wu LG, Westenbroek RE, Borst JG, Catterall WA, and Sakmann B.
Weber BH, Wutz K, Gutwillinger N, Ruther K, Drescher B, Sauer C,
Calcium channel types with distinct presynaptic localization couple differ-
J Neurophysiol • VOL 92 • NOVEMBER 2004 • www.jn.org
L-TYPE CALCIUM CHANNELS
entially to transmitter release in single calyx-type synapses. J Neurosci 19:
Zhang N and Townes-Anderson E. Regulation of structural plasticity by
726 –736, 1999.
different channel types in rod and cone photoreceptors. J Neurosci 22:
Xu W and Lipscombe D. Neuronal Ca(V)1.3alpha(1) L-type channels acti-
7065–7079, 2002.
vate at relatively hyperpolarized membrane potentials and are incompletely
Zhang Z, Xu Y, Song H, Rodriguez J, Tuteja D, Namkung Y, Shin HS, and
inhibited by dihydropyridines. J Neurosci 21: 5944 –5951, 2001.
Chiamvimonvat N. Functional roles of Ca(v)1.3 (alpha(1D)) calcium
channel in sinoatrial nodes: insight gained using gene-targeted null mutant
Yasuda R, Sabatini BL, and Svoboda K. Plasticity of calcium channels in
mice. Circ Res 90: 981–987, 2002.
dendritic spines. Nat Neurosci 6: 948 –955, 2003.
Zhuravleva SO, Kostyuk PG, and Shuba YM. Subtypes of low voltage-
Yue DT, Backx PH, and Imredy JP. Calcium-sensitive inactivation in the
activated Ca2⫹ channels in laterodorsal thalamic neurons: possible localiza-
gating of single calcium channels. Science 250: 1735–1738, 1990.
tion and physiological roles. Pfluegers Arch 441: 832– 839, 2001.
J Neurophysiol • VOL 92 • NOVEMBER 2004 • www.jn.org
Source: https://weifengxu.mit.edu/wp-content/uploads/2014/05/l-type-calcium-channels-the-low-down.pdf
La lógica de la sobredeterminación: hacia una radica- lización del análisis político Resumen. El presente artículo se plantea, desde una gramática posfundacionalista, radicalizar el análisis político proponiendo a la sobredeterminación, en tanto lógica subyacente a todo proceso de significación, como un concepto clave para poder disolver las fronteras que le ha impuesto la ciencia política canónica. Procurando avanzar en una conceptualización de la noción de sobredeterminación, este trabajo intenta mostrar la presencia de la lógica de la sobredeterminación en una serie de investigaciones empíricas realizadas desde un marco analítico posfundacionalista, en cada una de las cuales se ausenta una discusión teórica en torno a esta noción clave.
The Oncologist CME Program is located online at http://cme.theoncologist.com/. Symptom Management and Supportive Care The Assessment and Management of Delirium in Cancer Patients HIRLEY H. BUSH,a,b,c,d EDUARDO BRUERA aDepartment of Palliative Care & Rehabilitation Medicine, University of Texas M.D. Anderson Cancer