Ifes.edu.br
J Nanopart Res (2013) 15:1879DOI 10.1007/s11051-013-1879-8
Improved photodynamic action of nanoparticles loadedwith indium (III) phthalocyanine on MCF-7breast cancer cells
Carlos Augusto Zanoni Souto • Kle´sia Pirola Madeira • Daniel Rettori •Mariana Ozello Baratti • Letı´cia Batista Azevedo Rangel • Daniel Razzo •Andre´ Romero da Silva
Received: 1 April 2013 / Accepted: 16 July 2013Ó Springer Science+Business Media Dordrecht 2013
Indium (III) phthalocyanine (InPc) was
(1.8–7.5 lmol/L), incubation time (1–2 h), and laser
encapsulated into nanoparticles of PEGylated poly
power (10–100 mW) were studied on the photody-
(D,L-lactide-co-glycolide) (PLGA-PEG) to improve
namic effect caused by the encapsulated and the free
the photobiological activity of the photosensitizer. The
InPc. Nanoparticles with a size distribution ranging
efficacy of nanoparticles loaded with InPc and their
from 61 to 243 nm and with InPc entrapment efficiency
cellular uptake was investigated with MCF-7 breast
of 72 ± 6 % were used in the experiments. Only the
tumor cells, and compared with the free InPc. The
photodynamic effect of encapsulated InPc was depen-
dent on PS concentration and laser power. The InPc-loaded nanoparticles were more efficient in reducingMCF-7 cell viability than the free PS. For a light dose
C. A. Z. Souto A. R. da Silva (&)Federal Institute of Espı´rito Santo, Campus Aracruz,
of 7.5 J/cm2 and laser power of 100 mW, the effec-
Avenida Moroba´, 248, Moroba´, Aracruz,
tiveness of encapsulated InPc to reduce the viability
ES 29192-733, Brazil
was 34 ± 3 % while for free InPc was 60 ± 7 %.
Confocal microscopy showed that InPc-loaded nano-
particles, as well as free InPc, were found throughout
Biotechnology Program/RENORBIO, Health Sciences
the cytosol. However, the nanoparticle aggregates and
Center, Federal University of Espı´rito Santo, Vitoria,
the aggregates of free PS were found in the cell
ES 29040-090, Brazil
periphery and outside of the cell. The nanoparticles
aggregates were generated due to the particles con-
Department of Exact Sciences and Earth, Federal
centration used in the experiment because of the small
University of Sa˜o Paulo, Diadema, SP 09972-270, Brazil
loading of the InPc while the low solubility of InPccaused the formation of aggregates of free PS in the
M. O. BarattiDepartment of Cellular Biology, University of Campinas,
culture medium. The participation of singlet oxygen in
Campinas, SP 13083-863, Brazil
the photocytotoxic effect of InPc-loaded nanoparticleswas corroborated by electron paramagnetic resonance
experiments, and the encapsulation of photosensitizers
Department of Pharmaceutical Sciences,Federal University of Espı´rito Santo, Vitoria,
reduced the photobleaching of InPc.
ES 29040-090, Brazil
PLGA-PEG Indium
phthalocyanine Nanoparticles MCF-7 cells
Department of Physical Chemistry, Institute of Chemistry,University of Campinas, Campinas, SP 13083-970, Brazil
Photodynamic therapy
J Nanopart Res (2013) 15:1879
Since poly(lactide-co-glycolide) (PLGA) is the mostwidely used polymer in pharmaceutical products
Photodynamic therapy (PDT) is an important thera-
approved by the Food and Drug Administration
peutic option for treating oncology (O'Connor et al.
(FDA) due to their biocompatibility and biodegrad-
; Sharma et al. ; Triesscheijn et al. and
ability, this polymer has a long safety record (Blander
non-oncology (Allison et al. ; Calzavara-Pinton
and Medzhitov ; Mundargi et al. ). There-
et al. ; Qiang et al. ) diseases. This modality
fore, our group has been exploring PLGA to encap-
combines a photosensitizer, light and oxygen mole-
sulate photosensitizers (Silva et al. , ).
cules (Juzeniene et al. ; Ochsner ). After
Several works have shown the benefits of PEGy-
administration of the photosensitizer, the diseased
lation across a broad range of polymer molecular
tissue is illuminated with visible light. The irradiation
architectures and macromolecular assemblies in
leads to excitation of the PS to a singlet electronic state
increasing the circulation half-life of polymeric nano-
(S1), which can be deactivated to the triplet state (T1)
particles that facilitate more opportunities for the
by non-radioactive processes (intersystem crossing)
passage of nanoparticles from the systemic circulation
(Juzeniene et al. ). In this state, the PS can interact
into the disordered and permeable regions of tumor
with oxygen molecules or other biomolecules that are
vasculature (Cruz et al. ; Gref et al. ; Jokerst
present near the irradiated region, thus generating
et al. ). In addition, polyethylene glycol (PEG)
reactive species that can damage the neoplastic tissues
has been clinically validated for many different
(Bozzini et al. ; Juzeniene et al. Ochsner
applications, and is currently listed as ‘‘Generally
Recognized as Safe'' by the FDA. This makes the
Administration of the lipophilic PS is a challenge in
PEGylated polymer particularly attractive for use in
PDT because of the poor solubility of the molecules in
the encapsulation of a hydrophobic PS (Knop et al.
physiologically compatible solvent media (Korbelik
et al. To overcome this problem, various
Metallic phthalocyanines stand among the most
delivery strategies have been studied so far to preserve
promising photosensitizers due to their intense absorp-
the hydrophobic photosensitizer in the aqueous envi-
tion in the ‘‘photodynamic window'' (600–800 nm),
ronment (Bechet et al. Konan et al. ;
long triplet lifetimes, and large singlet oxygen quan-
Korbelik et al. ). Indeed, the development of drug
tum yields (Garcia et al. ; O'Connor et al.
delivery systems such as liposomes, micelles, and
Considering that several metallic phthalocyanines
nanoparticles could improve the unfavorable biodis-
have been used in clinical and preclinical trials in
tribution of free PS (i.e., improvement of photosensi-
oncology (Sekkat et al. ) and that the presence of
tizer pharmacokinetic properties, better targeting of
In(III) in the core of the photosensitizer structure
diseased tissues due to particle size using the enhanced
enhances the in vitro and in vivo photodynamic
permeability, and retention effect, association to
efficacy (Chen et al. ; Rosenfeld et al. ), we
serum proteins and specific activation of the PS
decided to study indium(III) phthalocyanine (InPc) as
through localized delivery of the PS) as well as
a photosensitizer. Unfortunately, the InPc has a strong
avoidance of aggregation and loss of phototoxic
hydrophobic character that promotes aggregation in
activity/fluorescence which should result in a better
high polarity media hindering their systemic admin-
therapeutic outcome (Acharya and Sahoo ; Ma-
istration and restricting clinical studies. However, the
eda et al. Sekkat et al. ; Soares et al.
encapsulation of InPc in nanoparticles can solve this
Polymeric nanoparticles have been prominent among
the other delivery systems because of their capacity to
The aim of this study was to encapsulate InPc in
release drugs at an experimentally predetermined rate
PLGA-PEG nanoparticles to improve the photobio-
over a prolonged period of time; maintain drug
logical activity of the photosensitizer. The photody-
concentration with therapeutically appropriate ranges
namic efficacy of nanoparticles loaded with InPc on
in circulation and within tissues; protect drugs from
MCF-7 human breast tumor cells was evaluated and
hepatic inactivation, enzymatic degradation and rapid
compared with free InPc. The particles were charac-
clearance in vivo, and due to their simple preparation
terized with respect to surface morphology, size, zeta
methods (Kamaly et al. ; Mundargi et al.
potential, InPc loading, and entrapment efficiency.
J Nanopart Res (2013) 15:1879
The effects of incubation time, InPc concentration,
method, the dispersion of an immiscible organic
and laser power were studied on the photocytotoxicity
solvent in an aqueous phase with high pressure vapor,
of encapsulated or free InPc. The degree of internal-
such as dichloromethane, is necessary. However, InPc
ization of InPc-loaded nanoparticles and of free InPc
is not soluble in dichloromethane. Thus, experiments
into cells was evaluated by confocal microscopy and
were performed to determine the best solvent to
afterward quantified by fluorescence. The formation of
solubilize InPc for preparing the PLGA-PEG nano-
singlet oxygen by the encapsulated and free photo-
particles loaded with InPc. An absorbance measure-
sensitizer was evaluated by electron paramagnetic
ment of the free InPc solution (1.0–5.0 lmol/L) was
resonance (EPR), and the influence of photobleaching
obtained in 1-methyl-2-pirrolidone (MP), ethyl acetate
on these results was studied.
(EA), dimethylformamide (DMF), and dimethylsulf-oxide (DMSO). Graphics of absorbance versus con-centration were obtained using the InPc absorbance
values at the maximum absorbance wavelength ineach solvent (682 nm for MP, 683 nm for DMF,
686 nm for DMSO, and 683 nm for EA) to determinethe absorptivity coefficient. The solvent with the
highest absorptivity coefficient was chosen as the best
PLGA ? 5 kDa PEG) was purchased from Evonik
solvent for InPc.
Rohm GmbH (Darmstadt, Hessen, Germany). Indiu-
For nanoparticle preparation, 50 mg of PLGA-
PEG were dissolved in 7.0 mL of dichloromethane
poly(vinyl alcohol) (PVA) (M
and 0.30 mg of InPc was dissolved in 3 mL of MP.
w 13–23 kDa, 89 %
The percentage of 30 % (v:v) of MP in the organic
phase was adequate to prevent InPc aggregation
(TritonÒ X-100), trypsin, ethylenediaminetetraacetic
when the InPc solution was mixed to PLGA-PEG
acid (EDTA), trypan blue, 2-amino-2-(hydroxy-
solution. The organic phase (InPc solution ? PLGA-
methyl)-1,3-propanediol (TrizmaÒ Base), sodium
PEG solution) was added slowly to 50 mL of an
pyruvate, penicillin, gentamicin, amphotericin B,
aqueous solution of PVA (1.5 % m/v) and ethanol
(5 % v/v), which was homogenized for 15 min at
(phalloidin-TRITC), and propidium iodide were pur-
24,000 rpm (UltraTurrax T25, IKA, Wilmington,
chased from Sigma Chemical Company (St. Louis,
NC, USA). Since PLGA-PEG is characterized by a
MO, USA). Dimethylsulfoxide (DMSO), ethanol,
low glass transition temperature (Tg) (30 °C) (Loch-
sodium chloride, potassium chloride, dibasic sodium
mann et al. ), the jacketed glass was connected
phosphate, monobasic potassium phosphate and para-
to an ultrathermostatic bath that maintained the
formaldehyde were obtained from Vetec Quı´mica
internal circulating water at 15 °C. The initial and
Fina Ltda (Duque de Caxias, RJ, Brazil). RPMI 1640
final temperatures of the solution were 20 ± 1 and
medium and fetal bovine serum were purchased from
26 ± 2 °C, respectively. The resulting emulsion was
Cultilab (Campinas, SP, Brazil). ProLongÒ Gold with
maintained in magnetic agitation for 24 h for
40,6 diamidine-2-phenylindol (DAPI) was obtained
evaporation of organic solvents. The particles were
from Invitrogen (Sa˜o Paulo, SP, Brazil). The water
recovered by centrifugation at 64,0009g for 22 min
used throughout the experiment was first bi-distilled
at 19 °C (Beckman J2-21, Beckman Instruments,
and then deionized (Millipore). All other chemicals
Fullerton, CA, USA), and washed 3 times with water
were of analytical grade and were used without further
to remove excess PVA and non-incorporated InPc.
(mannitol: particle mass ratio of 1:1) were frozenin liquid nitrogen and freeze-dried at 28 lmHg
Nanoparticle preparation
and—45 °C in a LioBras lyophilizer, model LIOTOPL101 (Sa˜o Carlos, SP, Brazil) for 2 days. Three
The particles were prepared using the emulsion/
independent formulations were prepared using this
evaporation technique (Jeffery et al. For this
J Nanopart Res (2013) 15:1879
Particle morphology and mean size
represent the mean ± standard deviation (SD) forthree independent preparations of nanoparticles.
The morphology of nanoparticles was ascertained byscanning electron microscopy (SEM; JSM-6360 LV,
Cultivation of cancer cells
JEOL, Tokyo, Japan). Approximately 2 mg of lyoph-ilized particles were dispersed in deionized water and
MCF-7 human breast tumor cells (Rio de Janeiro Cell
a droplet of this aqueous suspension was placed
Bank, Duque de Caxias, RJ) were cultured in an RPMI
directly onto a metallic stub. Samples air-dried over
1640 medium supplemented with 10 % fetal bovine
the stub were coated with Au using a MED 020 Bal-
serum, 1.0 mmol/L sodium pyruvate, 2.0 mmol/L
Tec coater (Balzers, Liechtenstein). The mean particle
L-glutamine, 14 U.I./mL penicillin, 10 lg/mL genta-
size was determined by dynamic light scattering using
micin, and 3.5 lg/mL amphotericin, being incubated
a NPA152 Zetatrac from Microtrac (York, PA, US).
at 37 °C in a humidified environment having 5.0 %CO2 (Sanyo, Bensenville, Il). After confluence wasreached, the cells were washed twice with a phos-
Determination of InPc content in the nanoparticles
phate-buffered saline (PBS) solution (1.8 mmol/LKH
An amount of lyophilized InPc-loaded nanoparticles
10.1 mmol/L Na2HPO4, 136.9 mmol/L
NaCl, 2.7 mmol/L KCl) and harvested with trypsin
(2.0 mg) without mannitol was dissolved in MP
(0.25 % m/v)-EDTA (0.02 % m/v) solutions. They
(2.0 mL) (a good solvent for both PLGA-PEG and the
were seeded at a density of 1.5 9 105 cells/well in
InPc). The InPc was quantified by UV–Vis spectroscopy
96-wells plates and allowed to grow for 48 h.
(Agilent Cary 50 Conc, Santa Clara, CA, USA) at682 nm using an analytical curve obtained with ten
Photocytotoxic activity of the encapsulated
different InPc concentrations. The PLGA-PEG polymer
did not cause interference at the selected wavelength.
InPc incorporation efficiency was calculated using Eqs.
The photocytotoxic effect of encapsulated and free
) and ). The determinations were carried out in
InPc on the viability of MCF-7 cells was determined
triplicate and their mean values are reported.
InPc loading ð%Þ
razolium bromide (MTT) assay. The culture medium
mass of photosensitizer in particles
was removed and 200 lL fresh RPMI medium,
mass of particles
without fetal bovine serum and containing either
InPc-loaded nanoparticles or free InPc, were added toeach well of a 96-wells plate so that the irradiation of a
Entrapment efficiency ð%Þ
well does not reach the neighboring wells containing
photosensitizer solution. The irradiation of the wells
Theoretical InPc loading
was done in the laminar flow cabinet. For theexperiments performed with free InPc, the phthalocy-anine was solubilized in MP solution (0.18 % volumeMP: volume RPMI). The final InPc extracellular
Zeta potential measurement of nanoparticles
concentration was 7.5 lmol/L. Then, the MCF-7 cells(1.5 9 105 cells/well) were incubated for 1–2 h at
The zeta potential of nanoparticles was measured using
37 °C. After the desired incubation time, the wells
dynamic light scattering technology (NPA152 Zeta-
were washed with PBS solution and a fresh culture
trac, Microtrac Instruments, York, US) joined with the
medium was added. Each well was irradiated with a
interaction of random Brownian motion with driven
light dose of 7.5 J/cm2 using a laser diode INOVA
electric field motion of particle suspensions. Typically,
665 nm of Laserline (Amparo, SP, Brazil). This laser
3 mg of the lyophilized nanoparticles were dispersed
has a current selector, allowing us to work with
in 10 mL of deionized water, followed by sonication
different powers. A laser power of 60 mW was used
for a period of 1 min. The measure was done two times
for the experiments performed to determine the
for each preparation. The zeta potential values
J Nanopart Res (2013) 15:1879
encapsulated InPc on cell viability. Immediately after
saline solution. Then 2.0 mL of culture medium
irradiation, the culture medium was removed from the
containing InPc-loaded nanoparticles or free InPc
well and 15 lL of MTT solution (5.0 mg/mL) were
(7.5 lmol/L) were added to each well. After 2 h of
incubated for 4 h with cells. After this period, 70 lL of
incubation, cells were washed three times with the
sodium dodecyl sulfate solution (10 % m:v) in HCl
culture medium to eliminate excess particles or free InPc
0.01 mol/L were incubated with cells to solubilize the
that was not entrapped by the cells and harvested with
formazan crystals. After 12 h, absorbance of the
trypsin-EDTA solution. The suspension was centrifuged
formazan was measured in 570 nm in each well using
at 5009g for 5 min and the cells were resuspended with
an ELISA plate reader (Bioclin MR-96-A, Belo
500 lL of TritonÒ X-100 solution (0.5 % m:v) in a
Horizonte, MG, Brazil) to determine cellular viability.
sodium hydroxide solution (0.2 mol/L) to permeabilize
The absorbance of treated cells and the control
the cellular membrane and expose the encapsulated and
(untreated cell) were used for determining the per-
free InPc. Subsequently, 1.5 mL of MP were added to
centage of cell viability (Eq. ).
solubilize the encapsulated and free InPc. The InPc in thecell extracts was determined spectrofluorimetrically,
absorbance of treated cells
% cell viability ¼
based on a previously constructed analytical curve using
absorbance of the control
a PerkinHelmer LS 55 fluorescence spectrometer (Wal-tham, MA, USA). The values presented are the
The photocytotoxicity of InPc-loaded nanoparticles
mean ± SD of three independent replicates. Statistical
and of the free InPc was also evaluated by varying:
analyses were carried out using the Student's t test with a
(i) InPc concentrations (1.8–7.5 lmol/L) and (ii) laser
significance level of p 0.05.
power (10–100 mW). All experiments were carried outin a dark room to prevent the influence of surrounding
Cellular localization of InPc using confocal
radiation on the photocytotoxic effect. Dark control
toxicity was examined through wells containing onlycells incubated with RPMI, or cells incubated with
MCF-7 cells (7.5 9 105 cells/mL) were seeded in
RPMI and InPc-loaded nanoparticles or with free InPc,
plastic Petri dishes (16 9 10 mm) containing a spher-
or cells incubated with RPMI and 0.18 % MP, or cells
ical slide ([ = 13 mm) immersed in RPMI 1640
incubated with RPMI and InPc-free nanoparticles. Light
medium complemented with 10 % fetal bovine serum.
control toxicity was also examined through wells
The Petri dishes were then incubated at 37 °C and 5 %
containing only cells incubated with RPMI and irradi-
of CO2 for 48 h. The culture medium was removed
ated by the laser diode 665 nm (light), or cells incubated
and 500 lL of RPMI medium containing InPc-loaded
with RPMI and 0.18 % MP and irradiated by the laser,
nanoparticles or free InPc (7.5 lmol/L) were added to
or cells incubated with RPMI and InPc-free nanoparticle
each dish. After 2 h of incubation, the cells were
and irradiated by laser diode. The equivalent concen-
rinsed twice with a phosphate-buffered saline solution
tration of PLGA-PEG nanoparticles loaded with InPc
to eliminate the encapsulated or free InPc which was
was used in the experiment performed with free InPc
not attached to the cellular culture. Then, the cells
nanoparticle. The results presented are the mean ± SD
were fixed with 4 % (m/v) paraformaldehyde solution
of three independent replicates. Statistical analyses were
for 20 min at room temperature, rinsed three times
carried out using the Student's t test with a significance
with PBS, and the slide was removed from the Petri
level of p 0.05.
dish. A TritonÒ X-100 solution (0.2 % m:v) inTrizma-buffered saline (TBS) solution (150 mmol/L
Quantification of internalized InPc
of NaCl and 50 mmol/L of TrizmaÒ base) was addedon the slide surface for 5 min to permeabilize the cells.
The encapsulated and free InPc internalized in MCF-7
The permeabilization was blocked by placing a
cells was quantified through the method described by
solution of bovine albumin (3 % m:v) in PBS on the
Win et al. (Win and Feng MCF-7 cells were
slide surface for 30 min at room temperature. Subse-
grown in a 12-wells plate at 37 °C for 48 h using an
quently, 100 lL of phalloidin-TRITC were placed
RPMI 1640 medium. After this period, the wells with
onto the slide for 30 min to visualize the cell
cells were washed twice with a phosphate-buffered
cytoskeleton. The slides were washed twice with
J Nanopart Res (2013) 15:1879
PBS to eliminate residual phalloidin-TRICT and
is detectable using EPR measurements (Lion et al
100 lL of a ProLongÒ Gold solution, containing
). Typically, TEMP solutions (50 mmol/L) in
40,6-diamidine-2-phenylindol (DAPI) were added to
RPMI 1640 medium, containing InPc-loaded nano-
stain the cell nucleus at room temperature. All
particles or free InPc (7.5 lmol/L), were added to a
experiments were carried out in a dark room to
cylindrical glass cell and afterward irradiated with a
prevent photodegradation of the probes. The slides
light dose of 3.0–7.5 J/cm2 and a laser power of
were examined under a Zeiss Confocal LSM 510
100 mW at room temperature (25 °C). The same
microscope (Carl Zeiss microimaging, Inc., Thorn-
irradiation system (a laser diode 665 nm) was used in
wood, NY) equipped with Argon (kex = 543 nm) and
this experiment. Because of the hydrophobicity of
Helium–Neon (kex = 633 nm) lasers. Phalloidin-
InPc, the free InPc stock solution was prepared in MP
TRICT was excited at 543 nm and its fluorescence
solvent. Thus, the EPR experiment with free InPc was
was selected with a BP 560–615 filter that passes
performed in culture medium containing 0.18 % (v:v)
radiation with wavelengths ranging from 560 to
of MP. EPR spectra were recorded with a JES-FR30
615 nm. The InPc was excited at 633 nm and its
JEOL spectrometer using a rectangular cavity after the
fluorescent emission was selected using an LP650
incident light dose. Experiments were also performed
filter that passes radiation with wavelengths higher
in the presence of 5.0 lmol/L of NaN3 (singlet oxygen
than 650 nm. The DAPI was excited using a mercury
suppressor) and 0.24 mmol/L of TweenÒ 20 (non-
lamp and no filter was used. Optical cross-sections
ionic surfactant). For assessing if singlet oxygen is
were obtained with a gradual increase of 1.57 and
generated by encapsulated InPc or by InPc molecules
0.79 lm in depth to evaluate the InPc-loaded nano-
that were released from nanoparticles into the culture
particles and free InPc distribution in the cells,
medium, the nanoparticles were incubated for 30 min
in the medium, after which, the medium was centri-
InPc-loaded nanoparticles were incubated for 2 h
fuged (24,0009g, 30 min) and the supernatant was
with only culture medium to evaluate if the nanopar-
irradiated in the presence of TEMP using a light dose
ticle could aggregate in the culture medium during the
of 7.5 J/cm2. Measurements were carried out at room
incubation time with MCF-7 cells. The experiment
temperature with the following instrument settings:
was also performed in water to evaluate if the culture
microwave power: 4 mW; microwave frequency:
medium could cause the aggregation of the particles.
9.41 GHz; field modulation frequency: 100 kHz; field
The same concentration of nanoparticle (1.28 mg/mL)
modulation amplitude: 1 G; time constant: 0.30 s; scan
incubated with MCF-7 cells was used in this exper-
time: 120 s; number of scans: 1; field center: 3375 G;
iment. The average size of nanoparticle and the
field width: 15 G. All experiments were carried out in a
percentage of particles with a certain average size
dark room to prevent the influence of surrounding
were measured in determined period during the
incubation time (2 h) using the light dynamic scatter-ing. The same procedure was performed in the culture
Free and encapsulated InPc photobleaching
medium to measure the size and percentage of freeInPc aggregates. In this case, the concentration of free
Stock solutions of free InPc were prepared in MP due
InPc was the same used in the experiment of irradi-
to the low solubility of the photosensitizer in water. To
ation of the cells (7.5 lmol/L). After 2.0 h of incuba-
obtain the desired concentration of InPc in the
tion, a TweenÒ 20 solution (0.24 mmol/L) was added
photobleaching assays (5.0 lmol/L), aliquots of the
to the nanoparticle or the free InPc solutions and the
stock solution were added to a photooxidation
size and percentage of particles were measured again.
medium composed of PBS solution and TweenÒ 20(0.24 mmol/L). The concentration of TweenÒ 20 was
EPR measurements for detection of singlet oxygen
(0.04 mmol/L) to reduce the formation of free InPc
Generation of singlet oxygen was detected by EPR
aggregates. The final percentage of MP in the photo-
spectroscopy using TEMP. The reaction between 1O2
oxidation medium was the same percentage used in the
and TEMP generates a stable nitroxide radical 2,2,6,6-
experiments performed with MCF-7 cells (0.18 %
tetramethyl-4-piperidone-N-oxide (TEMPONE) that
v/v). The photooxidation medium containing free InPc
J Nanopart Res (2013) 15:1879
solution were added to a quartz cuvette and irradiated
Results and discussion
with a light dose of 0.5–5.0 J/cm2 at room temperatureusing a laser diode 665 nm. The experiments were
Preparation and characterization of InPc-loaded
performed using several laser powers (1–60 mW).
The same conditions were used to irradiate the freeInPc solutions (5.0 lmol/L) prepared using MP from
Figure disclosed that MP was more efficient to
dilution of stock solution. In this experiment, the
solubilize the InPc compared with other studied
irradiated solution contained only InPc and MP. The
solvents since the absorbance of free InPc was the
InPc absorbance was monitored from 550 to 800 nm
highest in MP. The absorptivity coefficient for InPc
after each incident light dose. Then, a graphic of
in MP [(1.8 ± 0.1) 9 105 L/mol cm] was 15 times
relative absorbance intensity versus light dose was
higher than the smallest absorptivity obtained in
obtained at 682 nm for free InPc solution prepared in
AE [(1.2 ± 0.1) 9 104 L/mol cm] and increased
MP (without PBS solution and TweenÒ 20), and at
according to the following order of solvents: AE [(1.2
690 nm for the free InPc solution diluted in PBS
± 0.1) 9 104 L/mol cm] DMSO [(2.2 ± 0.4) 9
solution containing TweenÒ 20. The relative absor-
104 L/mol cm] DMF [(4.5 ± 0.7) 9 104 L/mol cm]
bance intensity was obtained by dividing the InPc
MP [(1.8 ± 0.1) 9 105 L/mol cm]. Therefore, we
absorbance intensity after light dose by absorbance
decided to use MP to dissolve InPc to prepare the InPc-
intensity before irradiation. This procedure was
loaded PLGA-PEG nanoparticles.
repeated for each laser power. Suspension of InPc-
Since InPc is not soluble in dichloromethane, we
loaded nanoparticles (with InPc concentration of
evaluated whether the mixture of InPc solution in MP
5.0 lmol/L) in PBS solution with 0.24 mmol/L of
with dichloromethane could cause changes in the InPc
TweenÒ 20 was also irradiated with the same incident
spectrum. Figure shows that the ratio of 30 % (v:v)
light doses and laser powers used in the photobleach-
of MP with 70 % (v:v) of dichloromethane did not
ing assays of the free InPc. After the light dose, the
change the InPc spectrum after 15 min of mixture
absorbance spectrum of encapsulated InPc was mea-
(time used for preparing the nanoparticles). The
sured from 550 to 800 nm and the graphic of relative
PLGA-PEG also did not change the InPc spectrum
absorbance intensity at 690 nm versus light doses was
when the polymer was dissolved in the mixture of MP
obtained. In order to conclude whether the encapsu-
and dichloromethane, and it did not show absorbance
lation of InPc decreases the photobleaching of the
from 300 to 800 nm (Fig. inset). The result
photosensitizer, the suspension was centrifuged at
revealed that this mixture of MP with dichloromethane
20,4009g for 20 min and the InPc-loaded nanoparti-
(30 and 70 % respectively) is efficient for maintaining
cles were recovered and dissolved in MP for three
the monomeric state of InPc molecules evidenced by a
particular situations: before the irradiation of the
sharp Q band, typical of a monomeric phthalocyanine
suspension, after the incident light dose of 5 J/cm2
complex (Stillman and Nyokong ). This is
with a laser power of 60 mW and after the same light
important because the aggregation decreases the
dose but with a laser power of 100 mW. Subsequently,
photodynamic efficacy of photosensitizer. Therefore,
the absorbance spectrum of InPc in MP was measured
this mixture was used to prepare the PLGA-PEG
and compared before and after incident light dose. All
nanoparticles loaded with InPc.
experiments were carried out in a dark room to prevent
The SEM image of nanoparticles (Fig. shows
the influence of surrounding radiation on InPc
that particle shapes are spherical and are relatively
homogenous in size. Dynamic light scattering results
Free InPc spectra were obtained in MP or in PBS
show that the average particle size was (127 ± 8) nm,
solution containing TweenÒ 20 for evaluation of the
and the particle sizes distribution ranged from 61 to
monomeric state of the InPc before the irradiation
243 nm, with 90 % of the nanoparticles smaller than
period carried out in the photobleaching experiments.
200 nm (images not shown). Particles smaller than
Absorbance measurements were also performed with
200 nm stay in the bloodstream longer due to the
and without TweenÒ 20 to evaluate the presence of
reduced recognition of these particles by the mono-
nanoparticle aggregates during the experiment with
nuclear phagocytic system (Bourdon et al. ; Hans
the encapsulated InPc.
and Lowman ; Konan et al. They also
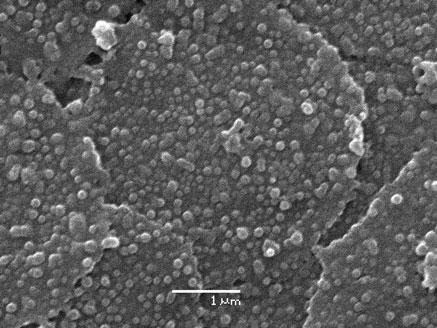
J Nanopart Res (2013) 15:1879
the cells (results not shown). Therefore, InPc-freenanoparticles, light and MP did not influence theresults obtained for cell viability of MCF-7 cellsincubated with InPc-loaded particles or the free InPc.
Effect of incubation time on phototoxicity
A significant decrease in cell viability was observed aftera light dose of 7.5 J/cm2 when the MCF-7 cells wereincubated with InPc-loaded PLGA-PEG nanoparticlesfor 1 h since the viability decreased from (100 ± 5) %(number of control cells) to (44 ± 4) % (cells ? nano/InPc ? light) (Fig. ). A similar decrease in cellviability was obtained when cells were incubated withInPc-loaded nanoparticles for 1.5 and 2 h and irradiatedby the same light dose since viability was reduced to(49 ± 9) % and to (47 ± 10) %, respectively, suggest-ing the photodynamic effect was not changed after 1 h ofincubation (Fig.
(100 ± 10) % (number of control cells) to (69 ± 6) %(cells ? free InPc ? light) only after 1.5 h of incubationand subsequent irradiation (Fig. ). The increase inincubation time of 1.5–2 h did not have any significanteffect on cell viability since viability was maintained at
Fig. 1 a Absorbance intensity versus InPc concentration in
(69 ± 4) %, suggesting the photodynamic effect was not
several solvents. The absorbance values were determined at the
changed after the incubation time of 1.5 h (Fig. ).
InPc maximum absorbance wavelength for each solvent
Neither encapsulated InPc nor free InPc caused cytotoxic
(682 nm for MP, 683 nm for DMF, 686 nm for DMSO and683 nm for EA). The solvent with the highest absorptivity
effects (without light) to MCF-7 cells under the same
coefficient was chosen as the best solvent for InPc. b Absorbance
conditions (Fig. ). Statistical analysis shown that the
spectra of InPc (5.0 lmol/L) dissolved in MP and in the mixture
encapsulated InPc was more efficient than free InPc in
of 30 % (v:v) of MP with 70 % of dichloromethane. The PLGA-PEG also did not change the InPc spectrum (inset) when thepolymer was dissolved in the mixture of MP and dichlorometh-ane, and it did not show absorbance from 300 nm to 800 nm
interact more efficiently with cellular membranes(Win and Feng The PLGA-PEG nanoparticlesexhibited an InPc entrapment efficiency of around(72 ± 6) %, a loading value of around (0.43 ±0.04) % and a zeta potential of -33.9 ± 3 mV.
Viability of MCF-7 cells in action involvingonly InPc-free nanoparticles, light and MP
The photocytotoxic effect on the viability of MCF-7cells after incubation and irradiation with InPc-freenanoparticles was not observed. This is consistent with
Fig. 2 SEM images of InPc-loaded PLGA-PEG nanoparticles,
PLGA-PEG biocompatibility. The light emitted by the
prepared by the emulsion/evaporation method using PVA as
laser and MP (0.18 % v:v) were also not cytotoxic to
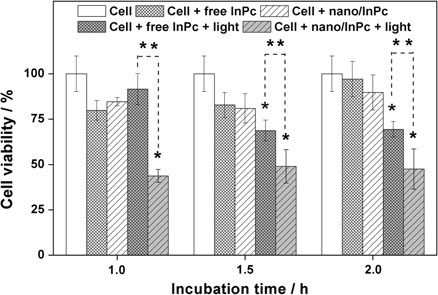
J Nanopart Res (2013) 15:1879
causing cellular death since less cell viability was
for a concentration of 1.8 lmol/L, subsequently to
obtained using InPc-loaded nanoparticles with 1 h
(63 ± 3) % (number of cells ? nano/InPc ? light)
[(44 ± 4) %], 1.5 h [(49 ± 9) %], or 2 h [(47 ±
for a concentration of 3.8 lmol/L and then to
10) %] of incubation and 7.5 lmol/L of InPc whether
(47 ± 6) % (number of cells ? nano/InPc ? light)
compared with the viability obtained using free InPc for
when the InPc concentration was 7.5 lmol/L (Fig.
1 h [(92 ± 9) %], 1.5 h [(69 ± 6) %], or 2 h [(69 ±
However, free InPc only reduced cell viability from
4) %] of incubation (Fig. ). It seems that encapsulated
(100 ± 6) % (number control of cells) to (71 ± 8) %
InPc was internalized faster than free InPc since the
(number of cells ? free InPc ? light) when the InPc
photocytotoxic effect was observed after an incubation
concentration was 7.5 lmol/L (Fig. ). The increase
time of 1 h for InPc encapsulated and after 1.5 h for free
of free InPc concentration to 30 lmol/L (results not
InPc. Since the reduction in cellular viability between 1.5
shown) did not change significantly the results
and 2 h was not significantly different for MCF-7 cells
obtained using the concentration of 7.5 lmol/L. The
incubated with InPc-loaded nanoparticles, or with free
ability of free zinc phthalocyanine (ZnPc) in reducing
InPc, after irradiation with a light dose of 7.5 J/cm2 and
the viability of A549 cells was also not changed after
laser power of 60 mW, an incubation time of 2 h was
concentration 17 lmol/L and the average viability
used for subsequent experiments.
was maintained at 62 % (Soares et al. ). FreeZnPc was not cytotoxic even using the concentration
Effect of InPc concentrations on phototoxicity
at 69 lmol/L (Soares et al. ). Probably theaggregate state of free InPc in the culture medium
The increase in encapsulated InPc concentration from
decreased the photodynamic efficiency of free InPc to
1.8 to 7.5 lmol/L increased the photocytotoxicity of
reduce the cell viability (as will be shown later). The
the nanoparticulate formulation since cell viability
encapsulated InPc in PLGA-PEG nanoparticles or the
decreased from (100 ± 5) % (number of control cell)
free InPc was not cytotoxic for MCF-7 cells in the
to (82 ± 3) % (number of cells ? nano/InPc ? light)
dark. The results shown that the InPc-loaded nano-particles were more photocytotoxic than the free InPcsince the cell viability was reduced from (82 ± 6) %(number of cells ? free InPc ? light) to (63 ± 3) %(number of cells ? nano/InPc ? light) when the InPcconcentration was 3.8 lmol/L, and from (71 ± 8) %(number of cells ? free InPc ? light) to (47 ± 6) %(number of cells ? nano/InPc ? light) using the con-centration 7.5 lmol/L (Fig. This is in agreementwith results obtained by other researchers who encap-sulated photosensitizers into polymeric nanoparticles(Konan et al. Zeisser-Labouebe et al. These results also revealed that free InPc photocyto-toxicity was not dependent on the range of studied InPcconcentration. Since the encapsulated and free InPc
Fig. 3 Percent of cell viability versus incubation time on
exhibit significant photocytotoxic effects at concentra-
cytotoxicity (without light) and photocytotoxicity (with light) of
tions of 7.5 lmol/L in experiments with light, this
InPc-loaded nanoparticles and free InPc. InPc concentration inInPc-loaded nanoparticles and in the aqueous cell culture media
concentration was used in subsequent assays.
was 7.5 lmol/L. The MCF-7 cells were incubated with InPc-loaded nanoparticles and free InPc for different times (from 1 to
Effect of laser power on InPc phototoxicity
2 h) and then irradiated with a light dose of 7.5 J/cm2 (light infigure legend) using a 665 nm laser diode with a power of 60mW. The InPc-free nanoparticles, the source light and MP were
The effectiveness of encapsulated InPc (Fig. ) in
not cytotoxic for cells (results not shown). Each data point is the
causing cellular death was influenced by laser power
mean ± SD of three values. (*) The differences of ‘‘cell'' vs.
since viability was reduced from (100 ± 9) % (num-
‘‘cell ? nano/InPc ? light'', or ‘‘cell'' vs. ‘‘cell ? free InPc ?
ber control of cells) to (64 ± 3) % (number of
light'' or (**) ‘‘cell ? free InPc ? light'' vs. ‘‘cell ? nano/InPc ?light'' were significant at p B 0.05
cells ? nano/InPc ? light) and then to (34 ± 3) %
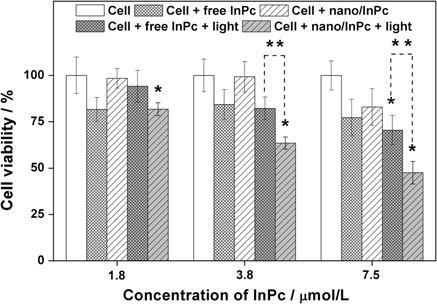
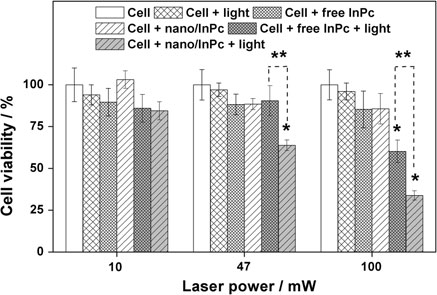
J Nanopart Res (2013) 15:1879
Fig. 5 Percent of cell viability versus laser power on cytotox-
Fig. 4 Percent of cell viability versus InPc concentrations in
icity (without light) and photocytotoxicity (with light) of InPc-
InPc-loaded nanoparticles and in aqueous cell culture media on
loaded nanoparticles and free InPc. InPc concentrations in InPc-
the cytotoxicity (without light) and photocytotoxicity (with
loaded nanoparticles and in aqueous cell culture media was
light) of InPc-loaded nanoparticles and free InPc. MCF-7 cells
7.5 lmol/L. MCF-7 cells were incubated with InPc-loaded
were incubated with InPc-loaded nanoparticles or free InPc for
nanoparticles or free InPc for 2 h and then irradiated using a
2 h and then irradiated with a light dose of 7.5 J/cm2 (light, in
665 nm diode laser with different powers (10–100 mW) (light,
figure legend) using a 665 nm diode laser with a power of
in figure legend). The InPc-free nanoparticles and MP were not
60 mW. The InPc-free nanoparticles, source light and MP were
cytotoxic for cells (results not shown). Each data point is the
not cytotoxic for cells (results not shown). Each data point is the
mean ± SD of three values. (*)The differences of ‘‘cell'' vs.
mean ± SD of three values. (*) The differences of ‘‘cell'' vs.
‘‘cell ? nano/InPc ? light'', or ‘‘cell'' vs. ‘‘cell ? free InPc?
‘‘cell ? nano/InPc ? light'', or ‘‘cell'' vs. ‘‘cell ? free InPc ?
light'' or (**) ‘‘cell ? free InPc ? light'' vs. ‘‘cell ? nano/InPc ?
light'' or (**) ‘‘cell ? free InPc ? light'' vs. ‘‘cell ? nano/InPc ?
light'' were significant at p B 0.05
light'' were significant at p B 0.05
(number of cells ? nano/InPc ? light) when cells
cells ? free InPc ? light) to (34 ± 3) % (number of
were incubated with InPc-loaded nanoparticles and
cells ? nano/InPc ? light) when laser power was
irradiated with a laser power of 47 and 100 mW,
respectively. The same dependence was not observedfor free InPc when laser power was increased from 47
Localization and uptake of the encapsulated
to 100 mW since viability was only reduced from
and free InPc into the MCF-7 cells
(100 ± 9) % (number control of cells) to 60 ± 7 %when laser power was 100 mW (Fig. ). Neither InPc-
Figure is the combination of four micrographies that
loaded nanoparticles nor free InPc caused the MCF-7
relate InPc green fluorescence, DAPI blue fluores-
cell death when a laser power of 10 mW was used. The
cence bound to the nucleus, phalloidin red fluores-
energy density emitted by laser was probably not
cence bound to the cytoskeleton, and a sum of these
efficient to observe the photodynamic effect when the
micrographies. InPc-loaded nanoparticles (Fig. a) as
laser power of 10 mW was used to excite the
well as free InPc (Fig. b) were localized throughout
the cytosol and in the perinuclear region, since the
10–47 mW was used to excite the free InPc. Cells
micrographs very clearly show the fluorescence emit-
not incubated with free or encapsulated InPc were
ted by InPc during excitation with a helium–neon
irradiated using different powers and no change was
laser. Micrographies also disclosed the presence of
observed in the cell viability, indicating that the laser
intense fluorescence of encapsulated InPc in the
energy did not fried the MCF-7 cells. Results show
micro-region of the cytosol near the peripheral surface
clearly that the encapsulated InPc was more effective
of the cell, suggesting the presence of aggregates of
in causing the death of MCF-7 cells than free InPc
PLGA-PEG particles loaded with InPc inside and in
since the cell viability was reduced from (90 ± 9) %
the periphery of MCF-7 cells (Fig. a). The PLGA-
(number of cells ? free InPc ? light) to (64 ± 3) %
PEG nanoparticles possess a highly hydrophilic sur-
(number of cells ? nano/InPc ? light) when laser
face coated with a PEG polymer. This characteristic
power was 47 mW, and from (60 ± 7) % (number of
could favor the interaction of nanoparticles with the
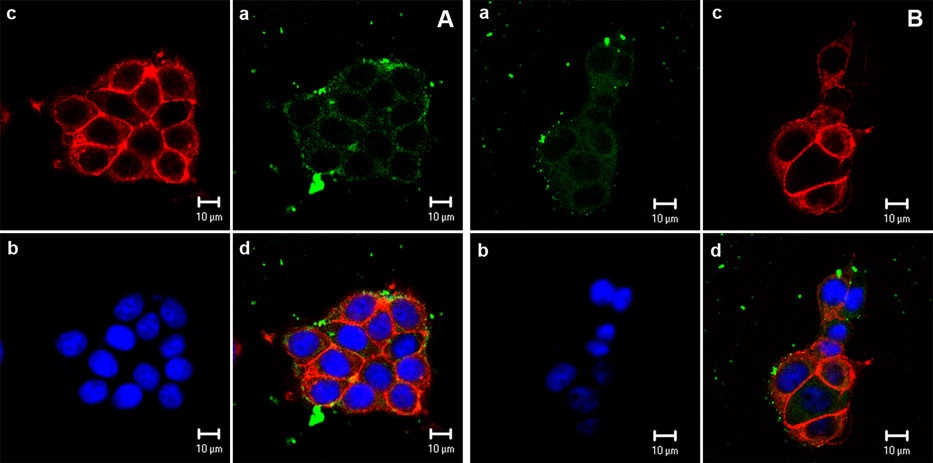
J Nanopart Res (2013) 15:1879
Fig. 6 Confocal micrographs of MCF-7 cells incubated for 2 h
(c) Phalloidin red fluorescence (argon laser kex = 543 nm)
with 7.5 lmol/L of a InPc encapsulated into nanoparticles or
bound to F-actin for staining the cytoskeleton and (d) a sum of
b free InPc. (a) InPc green fluorescence (helium–neon laser,
the three earlier micrographies. Similar results were obtained
kex = 633 nm); (b) DAPI blue fluorescence (mercury lamp,
with the other nanoparticle formulations and free InPc (results
kex = 365–372 nm) bound to DNA for staining the nucleus;
hydrophilic external membrane of the cell, causing a
(Fig. a). Experiments were also performed with water
concentration of PLGA-PEG nanoparticles in the
instead culture medium to evaluate if the aggregates
peripheral area of the cell. However, intense fluores-
were generated by interaction of nanoparticles with the
cence was also detected in the region away from the
culture medium (Fig. The results disclosed that
surface of the cell. Therefore, it was evaluated if the
the population of large aggregates of particles
aggregation could be caused due to the interaction
[(20 ± 2) %] was bigger in water than that obtained
between culture medium and nanoparticles. The
in the culture medium [(6 ± 1) %] after 2 h of
average size was measured at determined period
incubation, as well as the size was also larger since
during the incubation of nanoparticles only with
the average size of aggregates was (5950 ± 66) nm
culture medium for 2 h. One population of nanopar-
while in the culture medium the average size was
(1579 ± 16) nm (Fig. ). The presence of non-ionic
(132 ± 2) nm and other population [(2 ± 1) %] with
surfactant reduced the population and the size of the
an average size of (383 ± 13) nm were observed after
aggregates since the average size was reduced from
0.33 h of incubation, suggesting that the nanoparticle
(5950 ± 66) to (778 ± 17) nm and from (1579 ± 16)
aggregates were generated in the culture medium
to (1155 ± 17) nm when TweenÒ 20 (0.24 mmol/L)
(Fig. a). Large particles were not observed for
was added in water and in medium culture, respec-
incubation time smaller than 0.33 h.
tively. This result allows hypothesizing that the culture
The nanoparticle aggregates increased during the
medium helped to reduce the aggregates of InPc-
incubation time since the population of large particles
loaded nanoparticles. Probably, the aggregates of
increased from 0 to (6 ± 1) % and the population of
nanoparticles observed in the medium culture and in
the confocal micrographies were generated due to the
(94 ± 1) % after 2 h of incubation with the culture
nanoparticles concentration used in the experiments
medium, while the average size of large particles
because of the small loading of the InPc into the
increased from 0 to (1579 ± 16) nm and the small
nanoparticles. Unfortunately, the better values for
particles increased from (128 ± 10) to (151 ± 12) nm
loading were not possible because of the diffusion of
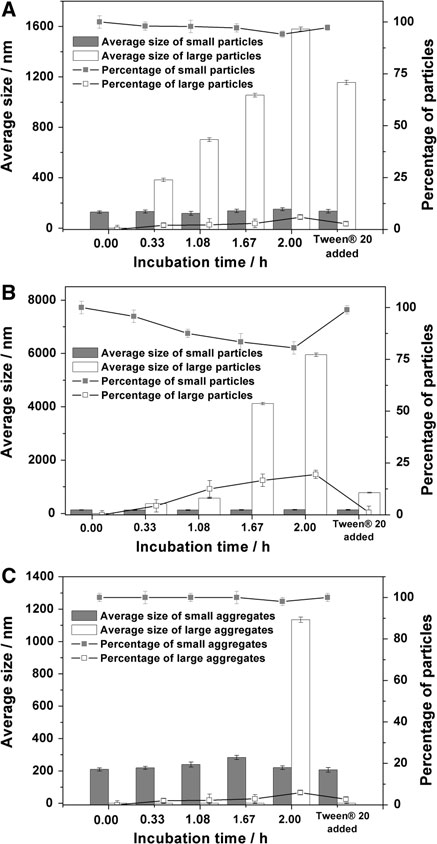
J Nanopart Res (2013) 15:1879
Studies have shown that hydrophobic photosensitizerstend to be localized in cytoplasmatic membrane (Moor; Ochsner ). However, the intense fluores-cence of free InPc was also detected in regions awayfrom of the cell. The free InPc solution in the culturemedium was also monitored by dynamic light scatter-ing to evaluate the presence of aggregates. Theoreti-cally, free InPc aggregates should not be detected if thesolution was homogenous, but when free InPc solutionprepared in MP was diluted in the culture medium, theaggregates were detected in initial of the experiment(Fig. c). The average size of small aggregatesincreased from (209 ± 10) to (283 ± 13) nm after1.67 h of incubation. One population [(1.9 ± 1) %] oflarge aggregates were detected after 2.0 h. However,the large aggregates were eliminated when TweenÒ 20(0.24 mmol/L) was added in medium culture suggest-ing that the large aggregates were reduced to smallersize. These results suggest that the molecules of freeInPc aggregated in culture medium due to the hydro-phobicity of InPc (as will be shown later). Therefore,the intense fluorescence detected outside of the cellwas emitted by aggregates of free InPc.
Fluorescence from InPc-loaded nanoparticles or
free InPc within the nucleus was not observed. Thus,there is no risk of photocarcinogenesis for survivingcells (Berg et al. ).
Cells incubated with nanoparticles of PLGA-PEG
without entrapped InPc did not present fluorescence(not
observed for nanoparticles loaded with InPc is onlydue to the photosensitizer. The fluorescence intensityof internalized InPc-loaded nanoparticles was higherthan that of the free InPc, suggesting that a greater
Fig. 7 Average size and percentage of InPc-loaded PLGA-
amount of encapsulated InPc was internalized in the
PEG nanoparticles incubated with a the culture medium andb water. Nanoparticles were incubated from 0 to 2 h and the
cells in comparison with free InPc. This hypothesis
average size and percentage of particles were measured in a
was evaluated by a quantification of the amount of
predetermined time. c Average size and percentage of aggre-
encapsulated and free InPc internalized in the cells.
gates of the free InPc incubated with culture medium was also
Although the results have corroborated the confocal
measured. After 2.0 h of incubation, a TweenÒ 20 solution(0.24 mmol/L) was added to the nanoparticle or the free InPc
micrographs (not shown), it was not possible to
solutions and the size and percentage of particles were measured
consider the quantification of the amount of encapsu-
again. Each data point is the mean ± SD of three values
lated and the free InPc into the cells since thenanoparticle aggregates and free InPc aggregates were
the InPc for the aqueous phase due to the interaction
not eliminated when the cells were rinsed with
with ethanol and PVA (results will be published).
phosphate-buffered saline solution. Probably the
Similarly, an intense fluorescence of free InPc
results reflected the amount of encapsulated and free
aggregates was localized in the peripheral region of
InPc localized inside and outside of the cell. Studies
MCF-7 cells (Fig. suggesting that free InPc
have shown that the encapsulation of photosensitizers
aggregates could be localized on the surface of cells.
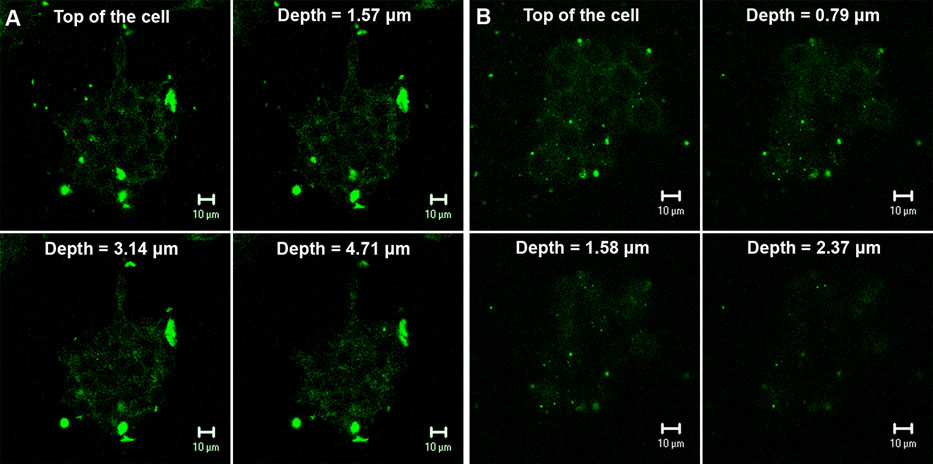
J Nanopart Res (2013) 15:1879
photosensitizer in the cell, a fact that will depend on
Hydrophobic photosensitizers tend to aggregate in
the photosensitizer, the carrier used in encapsulation
cell culture media due to the susceptibility of the
and the cell line studied (Nishiyama et al. ; Konan
hydrophobic skeleton to avoid contact with water
et al. The encapsulation of InPc and the low
molecules (Rosenthal The aggregation state
solubility of free InPc in the culture medium explain
hinders the efficacy of InPc by decreasing its bio-
the greater efficiency of encapsulated InPc in reducing
availability and limiting its capacity to absorb light
MCF-7 cell viability than free InPc.
(Bechet et al. Juzeniene et al. The
Optical cross-sections were obtained from the top
of the cells, with a gradual increase of 1.57 and0.79 lm in depth, respectively, for cells incubatedwith InPc-loaded nanoparticles or with free InPc toevaluate if encapsulated or free InPc were distributedwithin cells and/or near the cell surface. Figure confirmed the presence of InPc-loaded nanoparticlesinside MCF-7 cells since the fluorescence persisted inall optical sections (Fig. However, the distribu-tion of InPc-loaded nanoparticles in the cells was nothomogeneous since the fluorescence intensity fromnanoparticles was not uniform within these opticalsections. Similar results were observed for the distri-bution of free InPc into the MCF-7 cell (Fig. b). But,the intensity of green fluorescence from free InPc
Fig. 9 Free and encapsulated InPc absorbance and (inset)
within the optical sections was smaller than that
fluorescence spectra at a concentration of 7.5 lmol/L in RPMI1640 medium. Free InPc absorbance spectrum was also obtained
emitted by InPc-loaded nanoparticle, corroborating
in the same concentration but in MP. InPc was excited at 620 nm
with results obtained in Fig.
in both solutions
Fig. 8 Confocal micrographs of optical cross-sections taken
nanoparticles or b free InPc (7.5 lmol/L) for 2 h. InPc was
with a gradual increase of 1.57 and 0.79 lm in depth from the
excited with a helium–neon laser (kex = 633 nm)
top of the cell (top row) after incubation with a InPc-loaded
J Nanopart Res (2013) 15:1879
aggregation of free InPc in an RPMI 1640 medium
Generation of singlet oxygen by encapsulated
was also evaluated by absorbance and fluorescence
measurements (Fig. The absorbance spectrum offree InPc in an RPMI 1640 medium, compared with
The capacity of encapsulated and free InPc in generating
that obtained in MP, suggested that the InPc is
singlet oxygen was monitored by EPR measurements
aggregated in the culture medium since the wave-
using TEMP as a singlet oxygen trapping agent (Lion
length of maximum absorbance of free InPc was red
et al. ; Shutova et al ). The irradiation of the
shifted by 20 nm in the RPMI medium, with a
culture medium containing nanoparticles loaded with
concomitant decrease in absorbance intensity and a
InPc led to the generation of an EPR signal (Fig. a).
broadening of the Q band (400–800 nm) (Fig.
The triplet EPR signal is characteristic of a stable
corroborating with results obtained in Fig. c. The
nitroxide radical (TEMPONE) resulting from the reac-
molar absorptivity of the photosensitizer decreases
tion between singlet oxygen and TEMP (Lion et al.
significantly when large self-associated supra-struc-
Xu et al. ). The EPR signals increased
tures are formed, causing a decrease in absorbance
gradually with the increase in incident light from 3 to
intensity and, consequently, a reduction in the free
7.5 J/cm2, suggesting that singlet oxygen was gener-
InPc capability to absorb incident radiation (Reddi and
ated. This result is important because it shows the ability
Jori ). These large aggregates can also scatter
of encapsulated InPc to interact with molecular oxygen
incident radiation favoring a reduction in the absorp-
and that PLGA-PEG does not act as an impermeable
tion of free InPc. The InPc fluorescence also decreased
barrier. Before irradiation of TEMP, a small signal
(Fig. , inset) in the RPMI medium, in comparison
was detected due to the presence of TEMPONE
with the spectrum intensity in MP. The aggregates
have a reduced tendency for crossing plasmatic
The EPR signal did not change with an increase in
membranes and consequently have lower cellular
the light dose when the culture medium was irradiated
uptake. Thus, lower effectiveness of free InPc in
in the presence of free InPc (Fig. b). Experiments
causing MCF-7 cell death can be associated to the
were performed in the presence of a non-ionic
lipophilic character of InPc, which favors the aggre-
surfactant to evaluate the importance of aggregation
gation state in the aqueous culture medium. This
on generating singlet oxygen. The free InPc generated
reduces the capability of InPc to absorb incident
singlet oxygen in the presence of TweenÒ 20
radiation and decreases its cellular uptake, and its
(Fig. suggesting that the photosensitizer aggre-
subsequent ability to produce singlet oxygen.
gation reduced the efficacy of free InPc in generating
The absorbance and fluorescence spectra of the
singlet oxygen, and consequently the effectiveness in
encapsulated InPc, compared with that obtained in
decreasing the viability of MCF-7 cells. This result
MP, also suggested the presence of aggregates of
corroborates with the decrease in absorbance and
photosensitizer in the culture medium since the
fluorescence intensity of the free InPc spectrum.
absorbance and fluorescence intensities decreased
A similar experiment performed with InPc-loaded
and Q band suffered a broadening. However, the
nanoparticles and TweenÒ 20 caused an increase in
absorbance and fluorescence intensities of encapsu-
EPR signal intensity (Fig. suggesting the mole-
lated InPc were higher than that obtained to the free
cules adsorbed in the surface of nanoparticles could be
InPc. Especially, for absorbance spectrum of encap-
released into the culture medium in the presence of a
sulated InPc, the wavelengths considered in the
surfactant. Besides, InPc molecules adsorbed on the
conclusion were between 600–800 nm since wave-
nanoparticles surface could be responsible for the
lengths smaller than 600 nm presented higher scatter-
photodynamic effect of the encapsulated photosensi-
ing, causing an exponential profile in the spectrum. It
tizer on the death of MCF-7 cells. To evaluate this
is known that scattering is more intense in regions of
hypothesis, we incubated the InPc-loaded nanoparti-
smaller wavelength since the scattering intensity (SI)
cles with TEMP solution for 30 min. Before irradia-
is inversely proportional to the wavelength raised to
suspension was centrifuged and
the fourth power (SI a 1/k4) (Li et al. ). These
nanoparticles were discarded. The results revealed
results suggest that the aggregation of the encapsu-
that InPc-loaded nanoparticles were important for the
lated InPc was smaller than that the free InPc.
generation of singlet oxygen since the EPR signal was
J Nanopart Res (2013) 15:1879
Fig. 10 EPR spectra of TEMPONE generated by photooxida-
For assessing whether singlet oxygen is generated by encapsu-
tion of 2,2,6,6-tetramethylpiperidone (23 mmol/L) at room
lated InPc or by InPc molecules that were released from
temperature (25 °C) in the presence of a encapsulated and b free
nanoparticles into the culture medium, the nanoparticles were
InPc (7.5 lmol/L), in an RPMI 1640 culture medium. The
incubated for 30 min in the medium and after this time, the
generation of TEMPONE signal by c free and d encapsulated
medium was centrifuged before irradiation. Light source: laser
InPc was also monitored in the presence of 0.24 mmol/L of
diode 665 nm. Light doses of 7.5 J/cm2 and laser power of 100
TweenÒ 20 and 5.0 l mol/L of azide (singlet oxygen quencher).
mW were used in this experiment
not observed after the discarding of nanoparticles
Studies of free and encapsulated InPc
before the light dose. The TweenÒ 20 probably
reduced the nanoparticle aggregates, favoring photo-dynamic action of the greater amount of InPc-loaded
Phthalocyanines have the tendency to suffer photo-
nanoparticles, increasing the EPR signal.
bleaching (Bonnett and Martinez ). Thus, it is
An experiment performed in the presence of azide
possible that InPc photobleaching reduces the photo-
(a quencher of singlet oxygen) did not show the triplet
dynamic efficacy of free and encapsulated InPc in
EPR pattern for the encapsulated or the free InPc,
decreasing the MCF-7 cell viability or generating the
corroborating that the photodynamic effect was
EPR signal. For evaluating whether the encapsulated
caused by a generation of singlet oxygen. Control
and free InPc suffer photobleaching during the irra-
irradiations of only the culture medium or the free InPc
diation and whether the encapsulation decreases the
in water were carried out and no changes were
photobleaching of the photosensitizer, we monitored
observed (results not shown).
the absorbance spectrum of free and encapsulated InPc
J Nanopart Res (2013) 15:1879
Fig. 11 Free InPc photobleaching in a PBS solution and (inset)cin organic solvent. The decrease in relative absorbance of InPcsolution with concentration of 5.0 lmol/L was monitored at682 nm for the free InPc solubilized in MP, and at 690 nm forthe PBS solution containing the free InPc and 0.24 mmol/L ofTweenÒ 20. The solutions were irradiated with light doses from0.5 to 5.0 J/cm2 and laser power from 1 to 60 mW, using a laserdiode 665 nm. b Absorption spectra of free InPc (5.0 lmol/L) inMP and in PBS solution with TweenÒ 20, and of encapsulatedInPc (5.0 lmol/L) in PBS solution with and without TweenÒ 20.
The encapsulated InPc spectrum was also measured aftercentrifugation of the suspension for discarding the nanoparticlesloaded with InPc. c The photobleaching of encapsulated InPcwas also monitored in the PBS solution. (c, inset) Absorbancespectra of InPc-loaded nanoparticles (InPc concentration of5.0 lmol/L) dissolved in MP before and after irradiation with alight dose of 5 J/cm2 and a laser power of 60 and 100 mW. Afterthe light dose, the suspension of InPc-loaded nanoparticles inPBS solution was centrifuged at 20,4009g for 20 min, thesupernatant was discarded and the nanoparticles were dissolvedwith MP
during light doses (Fig. ). It is known that the RPMI1640 medium is a mixture of enriched salts with aminoacids, vitamins, glucose and glutathione. We showedthat RPMI 1640 can reduce the photobleaching ofphotosensitizers because some constituents of theculture medium quenched the singlet oxygen (Silvaet al. Thus, we decided to study InPc photo-bleaching in a PBS solution to prevent the influence ofthe culture medium on the experiment results.
The results show that the relative absorbance
intensity of free InPc (Fig. solubilized in PBSsolution and TweenÒ 20, decreases from 1.0 to 0.8with the increase in incident light from 0 to 5 J/cm2using a laser power of 60 mW. A smaller reduction inrelative absorbance intensity was obtained when thelaser power was 1 and 10 mW than when using apower of 60 mW. After a light dose of 5 J/cm2 therelative absorbance intensity was reduced from 0.94 to0.88 (Fig. when the laser power was increasedfrom 1 to 10 mW, and then to 0.80 when the laser
aggregation state of the free InPc in PBS solution
power was 60 mW. These results suggest that the free
was corroborated by an InPc absorbance spectrum
InPc was photobleached during the light doses. Free
characterized by a large Q band with small absorbance
InPc dissolved in MP (an organic solvent), irradiated
intensity (Fig. Thus, the aggregation state of free
with the same light doses and the same laser powers,
InPc favors a decrease in InPc photobleaching.
revealed higher photobleaching (Fig. a, inset) than
The results of the encapsulated InPc photobleach-
what was observed for free InPc in PBS solution
ing were inconclusive since relative absorbance
(Fig. since after a light dose of 5 J/cm2 the
intensity decreased and increased using the same light
relative absorbance intensity of free InPc in organic
doses and the same laser power as used for experi-
solvent was reduced from 0.93 to 0.47 when the laser
ments with free InPc (Fig. c). Probably, the light
power was increased from 1 to 60 mW. The
scattered by nanoparticles in PBS solution hampered
J Nanopart Res (2013) 15:1879
the measurements of absorbance. These results could
Therefore, encapsulated InPc suffers less photoble-
suggest that the encapsulated InPc was not photo-
aching than free InPc, which favors better photody-
bleached. This hypothesis was evaluated measuring
namic efficiency for encapsulated InPc in generating
the InPc absorbance spectrum after light dose on the
the EPR signal. The photobleaching of free InPc and
nanoparticle solution, subsequent centrifugation of the
its aggregation state in the culture medium probably
nanoparticle suspension, discarding of the superna-
reduces the efficacy of the free photosensitizer for
tant, and dissolution of the InPc-loaded nanoparticles
causing tumor cell death.
in MP. The intensity of the absorbance spectrumobtained by dissolving the InPc-loaded nanoparticlesin MP decreased after irradiation with a light dose of
5.0 J/cm2 and a laser power of 60 and of 100 mW(Fig. inset). This is an indication that encapsu-
This study demonstrated that the encapsulation of InPc
lated InPc was photobleached and that the oxygen can
into PLGA-PEG nanoparticles improves the photobi-
interact with the photosensitizer encapsulated in the
ological and photodynamic activity of the photosen-
nanoparticle. This outcome corroborates with EPR
sitizer. The photocytotoxicity of encapsulated InPc
results since encapsulation is not a barrier for photo-
was observed after 1 h of incubation with MCF-7
bleaching or for generating singlet oxygen. Figure
cells, and was depended on photosensitizer concen-
(inset) disclosed that the decrease in InPc absorbance
tration and laser power. The same result was not
intensity was smaller for encapsulated InPc than
observed for free InPc. InPc-loaded nanoparticles
observed for free InPc using the same light dose and
were more effective than free InPc in inducing MCF-7
laser power, since the relative absorbance of free InPc
cell death. The increase in phototoxicity of InPc-
after a light dose of 5 J/cm2 and a laser power of
loaded nanoparticles was related to the low solubility
60 mW was reduced from 1 (integral absorbance
of free InPc in the culture medium. The aggregation
intensity) to 0.79 (a decrease of 20 % in InPc
state and the photobleaching of free InPc reduce both
absorbance intensity) (Fig. while the relative
cellular uptake and its ability to produce singlet
absorbance of encapsulated InPc was reduced from 1
oxygen. Encapsulation is not a barrier to InPc for
to 0.93 (a decrease of 7 % in InPc absorbance
generating singlet oxygen. Moreover, encapsulation
intensity) (Fig. c, inset). Even a laser power of
decreases the photobleaching of InPc, favoring the
100 mW reduced the relative absorbance of encapsu-
augmentation of their photocytotoxicity.
lated InPc from 1 to 0.86 (a decrease of 14 % in InPc
We thank the Conselho Nacional de
absorbance intensity) (Fig. c, inset).
Desenvolvimento Cientı´fico e Tecnolo´gico (CNPq) and the
Absorbance measurement was also performed for
Federal Institute of Espı´rito Santo for financial support, the
encapsulated InPc in a PBS solution with and without
Instituto Nacional de Cieˆncia e Tecnologia de Fotoˆnica
surfactant before the light doses (Fig. The
Aplicada a Biologia Celular (INFABIC) for the confocalmicroscopy analysis and Prof. Geovane Lopes de Sena from
absorbance intensity of the encapsulated InPc spec-
Federal University of Espı´rito Santo for fluorescence analysis.
trum was higher in PBS solutions with surfactant thanthat measured without TweenÒ 20. After centrifuga-tion of the PBS solution containing the encapsulated
InPc for discarding the nanoparticles, the InPc absor-bance signal was quenched. Thus, the results suggest
Acharya S, Sahoo SK (2011) PLGA nanoparticles containing
the surfactant must reduce the aggregation of nano-
various anticancer agents and tumor delivery by EPR
particles increasing the InPc absorbance signal, cor-
effect. Adv Drug Deliv Rev 63:170–183. doi:
roborating with results shown in Fig. b, and that
Allison RR, Mota HC, Bagnato VS, Sibata CH (2008) Bio-
the increase in signal is not due to InPc molecules
nanotechnology and photodynamic therapy. State of the art
adsorbed in the surface of nanoparticles that could be
review. Photodiagn Photodyn Ther 5:19–28. doi:
released in the PBS solution. This is corroborating
Bechet D, Couleaud P, Frochot C, Viriot ML, Guillemin F,
with an increase in the EPR signal when TEMP was
Barberi-Heyob M (2008) Nanoparticles as vehicles for
irradiated in the presence of InPc-loaded nanoparticles
delivery of photodynamic therapy agents. Trends Bio-
and TweenÒ 20 (Fig.
technol 26:612–621.
J Nanopart Res (2013) 15:1879
Berg K, Western A, Bommer JC, Moan J (1990) Intracellular
nanoparticles: design, development and clinical transla-
localization of sulfonated mesotetraphenylporphines in a
human carcinoma cell line. Photochem Photobiol 52:481–
Knop K, Hoogenboom R, Fischer D, Schubert US (2010)
Blander JM, Medzhitov R (2006) Toll-dependent selection of
Poly(ethylene glycol) in drug delivery: pros and cons as
microbial antigens for presentation by dendritic cells.
well as potential alternatives. Angew Chem Int Ed
Nature 440:808–812. doi:
49:6288–6308. doi:
Bonnett R, Martinez G (2001) Photobleaching of sensitizers used
Konan YN, Gurny R, Allemann E (2002) State of the art in the
in photodynamic therapy. Tetrahedron 57:9513–9547.
delivery of photosensitizers for photodynamic therapy.
J Photochem Photobiol B 66:89–106. doi:
Bourdon O, Mosqueira V, Legrand P, Blais J (2000) A com-
parative study of the cellular uptake, localization and
Konan YN, Berton M, Gurny R, Allemann E (2003a) Enhanced
encapsulated in surface-modified submicronic oil/water
phenyl)porphyrin by incorporation into sub-200 nm nano-
carriers in HT29 tumor cells. J Photochem Photobiol B
particles. Eur J Pharm Sci 18:241–249. doi:
55:164–171. doi:
Bozzini G, Collin P, Betrouni N, Nevoux P, Ouzanne A, Puech
Konan YN, Chevallier J, Gurny R, Allemann E (2003b)
P, Villers A, Mordon S (2012) Photodynamic therapy in
Encapsulation of p-THPP into nanoparticles: cellular
urology: what can we do now and where are we heading?
uptake, subcellular localization and effect of serum on
Photodiagn Photodyn Ther 9:261–273. doi:
photodynamic therapy. Photochem Photobiol 77:638–644.
Calzavara-Pinton PG, Venturini M, Sala R (2005) A compre-
Korbelik M, Madiyalakan R, Woo T, Haddadi A (2012) Anti-
hensive overview of photodynamic therapy in the treatment
tumor efficacy of photodynamic therapy using novel
of superficial fungal infections of the skin. J Photochem
nanoformulations of hypocrellin photosensitizer SL052.
Photobiol B 78:1–6. doi:
Photochem Photobiol 88:188–193.
Chen Y, Zheng X, Dobhal MP, Gryshuk A, Morgan J, Dough-
erty TJ, Oseroff A, Pandey RK (2005) Methyl pyropheo-
Li K, Ma CQ, Liu Y, Zhao FL, Tong SY (2000) Rayleigh light
phorbide-a analogs: potential fluorescent probes for the
scattering and its applications to biochemical analysis. Chin
peripheral-type benzodiazepine receptor. Effect of central
Sci Bull 45:386–394.
metal in photosensitizing efficacy. J Med Chem 48:3692–
Lion Y, Delmelle M, van de Vorst A (1976) New method of
detecting singlet oxygen production. Nature 263:442–443.
Cruz LJ, Tacken PJ, Fokkink R, Figdor CG (2011) The influence
of PEG chain length and targeting moiety on antibody-
Lochmann A, Nitzsche H, von Einem S, Schwarz E, Mader K
mediated delivery of nanoparticle vaccines to human
(2010) The influence of covalently linked and free poly-
dendritic cells. Biomaterials 32:6791–6803. doi:
ethylene glycol on the structural and release properties of
Garcia AM, Alarcon E, Munoz M, Scaiano JC, Edwards AM,
Lissi E (2011) Photophysical behavior and photodynamic
Maeda H, Wu J, Sawa T, Matsumura Y, Hori K (2000) Tumor
activity of zinc phthalocyanines associated to liposomes.
vascular permeability and the EPR effect in macromolec-
ular therapeutics: a review. J Control Release 65:271–284.
Gref R, Minamitake Y, Peracchia MT, Trubetskoy V, Torchilin
Moor ACE (2000) Signaling pathways in cell death and survival
V, Langer R (1994) Biodegradable long-circulating poly-
after photodynamic therapy. J Photochem Photobiol B
meric nanospheres. Science 263:1600–1603.
Mundargi RC, Babu VR, Rangaswamy V, Patel P, Aminabhavi
Hans ML, Lowman AM (2002) Biodegradable nanoparticles for
TM (2008) Nano/micro technologies for delivering mac-
drug delivery and targeting. Curr Opin Solid State Mater
romolecular therapeutics using poly(D, L-lactide-co-gly-
Sci 6:319–327.
colide) and its derivatives. J Control Release 125:193–209.
Jeffery H, Davis SS, O'Hagan DT (1991) The preparation and
characterization of poly(lactide-co-glycolide) microparti-
Nishiyama N, Nakagishi Y, Morimoto Y, Lai P, Miyazaki K,
cles, I. Oil-in-water emulsion solvent evaporation Int J
Urano K, Horie S, Kumagai M, Fukushima S, Cheng Y,
Pharm 77:169–175. doi:
Jang W, Kikuchi M, Kataoka K (2009) Enhanced photo-
Jokerst JV, Lobovkina T, Zare RN, Gambhir SS (2011) Nano-
dynamic cancer treatment by supramolecular nanocarriers
particle PEGylation for imaging and therapy. Nanomed
charged with dendrimer phthalocyanine. J Control Release
6:715–728. doi:
133:245–251. doi:
Juzeniene A, Nielsen KP, Moan J (2006) Biophysical aspects of
O'Connor AE, Gallagher WM, Byrne AT (2009) Porphyrin and
photodynamic therapy. J Environ Pathol Toxicol Oncol 25:
nonporphyrin photosensitizers in oncology: preclinical and
clinical advances in photodynamic therapy. Photochem
Kamaly N, Xiao Z, Valencia PM, Radovic-Moreno AF, Far-
Photobiol 85:1053–1074.
okhzad OC (2012) Targeted polymeric therapeutic
J Nanopart Res (2013) 15:1879
Ochsner M (1997) Photophysical and photobiological processes
prostate tumour cells. J Photochem Photobiol B 94:101–
in the photodynamic therapy of tumours. J Photochem
Photobiol B 39:1–18. do
Silva AR, de Oliveira AM, Augusto F, Jorge RA (2011) Effects
Qiang YG, Zhang XP, Li J, Huang Z (2006) Photodynamic
of preparation conditions on the characteristics of PLGA
therapy for malignant and non-malignant diseases: clin-
ical investigation and application. Chin Med J 119:
phenylporphyrinato)indium(III). J Nanosci Nanotechnol
11:5234–5246. doi:
Reddi E, Jori G (1988) Steady-state and time-resolved spec-
Soares MV, Oliveira MR, dos Santos EP, Gitirana LB, Barbosa
troscopic studies of photodynamic sensitizers: porphyrins
GM, Quaresma CH, Ricci-junior E (2011) Nanostructured
and phthalocyanines. Rev Chem Intermed 10:241–268.
delivery system for zinc phthalocyanine: preparation,
characterization, and phototoxicity study against human
Rosenfeld A, Morgan J, Goswami LN, Ohulchankyy T, Zheng
lung adenocarcinoma A549 cells. Int J Nanomed 6:227–
X, Prasad PN, Oseroff A, Pandey RK (2006) Photosensi-
tizers derived from 132-oxo-methyl pyropheophorbide-a:
Stillman MJ, Nyokong T (1989) Chemical fixation and photo-
enhanced effect of indium(III) as a central metal in vitro
reduction of carbon dioxide catalyzed by metal phthalo-
and in vivo photosensitizing efficacy. Photochem Photo-
cyanine derivatives. In: Leznoff CC, Lever ABP (eds)
biol 82:626–634.
Phthalocyanines: properties and applications, vol 1.
Rosenthal I (1991) Phthalocyanines as photodynamic sensitiz-
chapter 3. Wiley, New York
ers. Photochem Photobiol 53:859–870
Triesscheijn M, Baas P, Schellens JHM, Stewart FA (2006)
Sekkat N, van den Bergh H, Nyokong T, Lange N (2012) Like a
Photodynamic therapy in oncology. Oncol 11:1034–1044.
bolt from the blue: phthalocyanines in biomedical optics.
Molecules 17:98–144.
Win KY, Feng SS (2005) Effects of particle size and surface
Sharma SK, Mroz P, Daı´ T, Huang Y, Denis TGS, Hamblin MR
coating on cellular uptake of polymeric nanoparticles for
(2012) Photodynamic therapy for cancer and for infections:
oral delivery of anticancer drugs. Biomaterials 26:2713–
what is the difference? Israel J Chem 52:691–705.
Xu S, Chen S, Zhang M, Shen T (2003) Synthesis, character-
Shutova T, Kriska T, Nemeth A, Agabekov V, Gal D (2000)
ization and photodynamic activity of phenmethylamino-
Physicochemical modeling of the role of free radicals in
demethoxy-hypocrellin B. J Photochem Photobiol B
photodynamic therapy. Biochem Biophys Res Commun
Zeisser-Labouebe M, Lange N, Gurny R, Delie F (2006)
Silva AR, Inada NM, Rettori D, Baratti MO, Vercesi AE, Jorge
Hypericin-loaded nanoparticles for the photodynamic
RA (2009) In vitro photodynamic activity of chloro
treatment of ovarian cancer. Int J Pharm 326:174–181.
Source: http://ifes.edu.br/images/stories/files/noticias/2013/08_Agosto/Artigo_publicado_Andre_Romero.pdf
CORNERSTONE RESEARCH FINANCIAL AND ECONOMIC CONSULTING AND EXPERT TESTIMONY The Economics of Irreparable Harm in Pharmaceutical Patent Litigation Rahul Guha, Cornerstone ResearchMaria Salgado, Cornerstone Research TABLE OF CONTENTS The Hatch-Waxman Act greatly simplifies the process of obtaining FDA approval for a generic drug. Under the Act, a generic company need only file an Abbreviated New Drug
iPF670 shown with stand Designed for low-volume printing, these printers offer affordable AVAILABLE WORKFLOW SOLUTIONS solutions that can support a variety • Mobile printing app*• Direct Print & Share** of applications including: posters, • PosterArtist Lite presentations, technical documents, • Print Plug-in for Microsoft Office®, and more!