Team.fh-kl.de
Microchim Acta (2009) 164:395–404DOI 10.1007/s00604-008-0073-7
Oxygen plasma-treated gold nanoparticle-based field-effectdevices as transducer structures for bio-chemical sensing
Jenny Gun & Dan Rizkov & Ovadia Lev &Maryam H. Abouzar & Arshak Poghossian &Michael J. Schöning
Received: 2 April 2008 / Accepted: 30 May 2008 / Published online: 4 July 2008
# Springer-Verlag 2008
Abstract EIS (electrolyte-insulator-semiconductor) sensors
based on the functionalization of uncoated gold nano-particles supported on a Si/SiO2 structure are presented.
Since they were first introduced to the sensor arena by
Oxygen plasma etching at moderate power (<200 W)
Brust et al. functionalized gold and precious metal
provides a convenient and efficient way to remove organic
nanoparticles have been increasingly used to enhance
capping agents from the gold nanoparticles without
electrochemical and photometric sensing applications
significant damage. Higher power intensities destroy the
Their high surface area, easy functionalization, high electric
linkage between the SiO2 and the gold nanoparticles, and
conductivity, high stability and corrosion resistance, and
some of the gold nanoparticles are removed from the
their pronounced plasmon resonance band in the visible
surface. The flat-band potential shift, i.e. the pH depen-
range as well as sensitivity to aggregation are amongst their
dence of the gold-coated EIS sensors is similar (33 mV/
most attractive features. However, despite the popularity of
pH) to the uncoated EIS pH-sensor. Lead, penicillin and
gold nanoparticles in electrochemical and photoelectro-
glucose sensors were prepared by immobilization of β-
chemical sensing there are only a few articles describing the
cyclodextrin, penicillinase and glucose oxidase by various
use of metal nanoparticle-based field-effect sensors. A
notable exception is the work of Willner's laboratory to detect neurotransmitters and DNA by modified gold
Keywords Field-effect device . Gold nanoparticles .
nanoparticle-coated alumina gate field-effect transistors
Functionalization . EIS sensor . Penicillin . Glucose . Lead
(FET). Willner's FET sensors utilize gold nanoparticlesembedded in a polymeric film (polyethyleneimine), whichhinders the accessibility of analytes to the functionalizedsurface of the nanoparticles. Presumably, the capping agentused to prevent aggregation of the nanoparticles in the
J. Gun : D. Rizkov : O. Lev (*)Institute of Chemistry, The Hebrew University of Jerusalem,
solution phase also limits the amount of the selective
recognition elements that can be bound on the gold
Jerusalem IL-91904, Israel
nanoparticles. This drawback deters to some extent
researchers from utilizing nanoparticles as a vector for
incorporation of desirable functionalities in FET sensors.
Institute of Nano- and Biotechnologies (INB),
In this article, we demonstrate a strategy to remove the
Aachen University of Applied Sciences, Campus Jülich,
protective capping agent moieties by oxygen plasma
treatment. Oxygen plasma ashing involves RF (radio
DE-52428 Jülich, Germany
frequency) excitation of pure oxygen gas under an electric
M. H. Abouzar A. Poghossian M. J. Schöning (*)
field to give active oxygen species that effectively oxidize
Research Centre Jülich,
(ash) carbonaceous materials. The ash and residues are
Institute of Bio- and Nanosystems (IBN2),
removed by mechanical vacuum pumping. The process is
DE-52425 Jülich, Germanye-mail:
[email protected]
routinely used in electronic industries either under low
J. Gun et al.
voltage to clean surfaces or under high power conditions
penicillinase (EC 3.5.2.6., Bacillus cereus from Sigma) with
(>100 W) to etch away undesirable polymeric films and
2,540 U/mg activity, penicillin, D-glucose, horseradish
photoresists. Here, we adopt the same setup to completely
peroxidase, HRP (type II), and o-dianisidine dihydrochloride
remove the capping agents off the gold nanoparticles, and
were purchased from Sigma-Aldrich.
to eliminate the thiol films that bind the gold nanoparticlesto the surface. As far as we know such a process was never
Preparation of gold sol Self-stabilized gold sol in toluene
reported before in the context of sensing applications. The
was prepared by a slightly modified Brust procedure
process was extensively used by Boyen and coworkers
Fifty milliliters of 50 mM tetraoctylammonium bromide in
for the removal of polymer coatings from gold nano-
toluene was added to a 200 ml Erlenmeyer containing
particles at 50 W oxygen plasma. Raiber et al. and
25 ml of a 40 mM aqueous solution of HAuCl4 and stirred
Hesse and Creighton recently demonstrated that self-
with a magnetic stirrer for 20 min. Then, the colorless
assembled monolayers of alkanethiolates and other thiolates
aqueous phase was separated in a separation funnel and
can be effectively etched off gold surfaces by either
discarded. The orange colored organic phase was returned
hydrogen or oxygen plasma etching. The authors showed
to an Erlenmeyer, and 25 ml of freshly prepared water
that the treatment results in an Au2O3-covered surface. In
solution containing 0.4 g of NaBH4 was added under
our case, the residual gold oxide is removed by alcohol
vigorous agitation. After the mixture was stirred for 2 h, the
reduction, leaving an organic-free sensing element. Due to
dark ruby organic phase was isolated and subsequently
its compatibility with the electronic industry, for a future
washed in aqueous 0.1 M H2SO4, then in 1 M Na2CO3, and
practical application of nanoparticle-based field-effect
finally in water. The organic phase was then dried by
devices, this technique was preferred over other well-
MgSO4 and filtered through a 0.45 μm PTFE syringe filter
established wet chemistry processes (e.g., Pirahna, ozona-
(MetaChem Technologies Inc., Torrance, CA;
tion, electrochemical etching) for removal of gold thiolate
The resulting sol contained approximately
12 mM gold with a mean particle diameter of 6–8 nm. The
Four different key experiments have been performed in
light absorption peak of the solution was at 523 nm
order to exemplify the use of plasma-etched gold nano-
wavelength (the optical density was 2.5–3.3 in a 2 mm3
cell) [The gold colloid solution was stored in 4 °C, no
devices: pH sensing by bare gold nanoparticle-modified
degradation was observed in half a year's time.
surfaces, lead ion-sensing by methylamine β-cyclodextrin-modified gold nanoparticles, penicillin and glucose sensingby adsorbed and covalently bonded enzymes (glucose
Substrate preparation and gold deposition
oxidase and penicillinase, respectively). Currently, the mainadvantage of using gold modified EIS stem from their
Sensor preparation and cleaning Al–Si–SiO2 structures
convenient use, but in a forthcoming publication we shall
(300 nm aluminum film as a rear-side contact layer, p-Si,
demonstrate that the same approach can be used to enhance
ρ=5–10 Ωcm, 30 nm dry thermally grown SiO2) were cut
glucose electrosensing
into pieces of 10 × 10 mm2 and washed for 5 minsequentially in an ultrasonic bath containing the followingsolvents: acetone, propanol, water, 2% Hellmanex II
Experimental section
(Hellma, solution, and in distilledwater once more. The cleaned sensors were then dried with
Reagents and materials
N2, and treated with O2 plasma at 250 W for 10 s. (TePLa-100 E, Technics Plasma, Germany). The oxygen partial
All chemicals were of reagent grade and were used as
pressure was 1.42 mbar. The preliminary tests showed that
a treatment with O2 plasma for a shorter time or with lower
95%, and hydrogen tetrachloroaurate were from Aldrich
power resulted in an inhomogeneous surface, imperfect
), toluene and DMSO were
subsequent silanization and eventually yielded poor gold
from J.T. Baker ), and sodium
borohydride was from Merck ().
Water was deionized (conductivity <0.1 μS) by a Seradest
Sensor silanization and gold modification The sensor
SD 2000 system (). For the
silanization procedure was similar to the reported protocol
pH measurements, technical buffer solutions (Titrisol,
Dry sensor substrates were exposed to a freshly
Merck) in the concentration range from pH 4 to pH 10 were
prepared solution of MPTMOS in toluene (10 vol%) at
used. Glucose oxidase (GOx; EC 1.1.3.4, type VII, from
20 °C for 1 h, rinsed five times with toluene, then three
Aspergillus niger) with an activity value of 121 U/mg,
times with isopropanol, and finally rinsed thoroughly with

Oxygen plasma-treated gold nanoparticle-based field-effect devices
acetone. In order to stop further undesirable hydrolysis and
thoroughly washed with distilled water and stored in
condensation reactions in the newly formed films, the
4.5 mM PBS buffer, pH 7.5, when not in use.
sensors were washed for 10 min in 1 mM aqueous aceticacid solution and then rinsed with water and dried. A gold
Surface characterization by ellipsometry, contact-angle
nanoparticle monolayer was deposited on the silanized
measurements, light-reflectance spectroscopy, and XPS
samples by immersion of the sensor in toluene–gold sol for
Thickness and homogeneity of the silane layer were
12 h. The sensors were then washed in toluene, isopropanol,
characterized by imaging ellipsometry (IE) (EP3, Nanofilm
acetone, and water.
Germany) equipped with a frequency doubled Nd:YAG laser(532 nm) and 60° incident angle. The imaging was
Sensor treatment with oxygen plasma The sensor treatment
conducted over an image area of 0.1 mm2 with a lateral
with O2 plasma was done always for 10 s in microwave
resolution of 2 μm. Ellipsometry was used to control the
plasma. The oxygen partial pressure in nitrogen atmosphere
thickness of the organosilane layer after every silanization
was 1.42 mbar. The plasma power was a subject of the
procedure. Optimal sensor performance was achieved when
additional studies described below.
the thickness of the organosilane layer was 1.6–2.0 nm,which is equivalent to the thickness of two monolayers. The
β-cyclodextrin (β-CD)-modified sensor Aminomethyl β-
contact angle was measured with an OCA20 CA system
cyclodextrin was synthesized according to ref. ]. The
(Dataphysics, Germany) utilizing the Sessile drop method
purity of the product was verified by H-NMR and
with 5 μl Milli-Q distilled water. Each substrate was dried
elemental analysis. The gold-modified surface was treated
with nitrogen before the measurement. Light reflectance of
with O2 plasma (10 s, 200 W) and then immersed in ethanol
the gold-coated sensors was measured using a Cary 1E UV–
overnight. Then, the sensor was left for 24 h in a water
vis spectrophotometer (Varian) operated in the reflectance
solution of aminomethyl β-cyclodextrin (50 mg/ml). The
mode. SEM (scanning electron microscopy) images were
sensor was ready for use immediately after careful water
performed by Zeiss Gemini 1550 at 60°. XPS (X-ray
wash to remove free aminomethyl β-CD.
photoelectron spectroscopy) measurements were done usingPHI 5600 equipped with a monochromatic AlKα radiation
Modification of sensor with penicillinase Two methods
were used to prepare the penicillin sensors:
1. Physical adsorption—3 mg of penicillinase were
Electrochemical measurement setup
dissolved in 1.5 ml of PBS buffer (pH 7.5, 20 mM);0.15 ml of this solution was pipetted onto the surface of
The schematic cross-section of the EIS sensor and the
the sensor and evaporated under nitrogen. The sensor
measurement set-up is presented in Fig. The EIS sensor
was washed with distilled water and kept in a
was mounted into a home-made measuring cell, sealed by
refrigerator when not in use.
an O-ring and contacted on its front side by the electrolyte
2. Chemical coupling—The gold nanoparticle-modified
and on its rear side by a gold-plated pin. An Ag/AgCl
sensor was washed twice with water, twice with DMSO
electrode (3 M KCl, Metrohm) that was used as a reference
and then left for 6 h in 4 mg/ml dithiobis(succinimy-
electrode was mounted from the top side. The side walls
dylpropionate) (DSP, from Pierce, Rockford, IL; ) in DMSO solution according tothe procedure of Pierce []. It was then washed twotimes with DMSO, two times with water and transferred
into 2.1 mg/ml of penicillinase in PBS buffer (pH 7.5,
20 mM) for overnight storage at 4 °C. Next, the sensorswere thoroughly washed with distilled water and stored
in 4.5 mM PBS buffer, pH 7.4 when not in use.
Modification of the sensor with glucose oxidase The gold
nanoparticle-modified sensor was washed two times with
water, two times with DMSO and left for 6 h in 4 mg/mlDSP in DMSO. It was then washed two times with DMSO,two times with water and transferred into 4.9 mg/ml ofGOx (Aldrich, 121 U/mg,) in NH4Ac 4.5 mM buffer,
Fig. 1 Schematic cross-section of the EIS sensor modified with gold
pH 6.8 for overnight storage at 4 °C. Next, the sensors were
nanoparticles and measurement setup. RE reference electrode
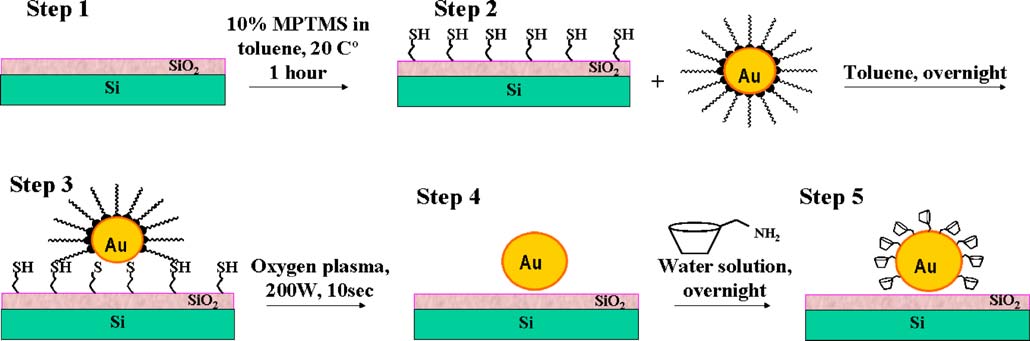
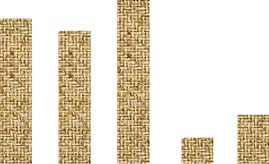
J. Gun et al.
Fig. 2 Sensor preparation: step 1 bare Si/SiO2 sensor chip, step 2 silanization, step 3 gold colloid deposition, step 4 oxygen plasma treatment,step5 surface modification of gold nanoparticles
and backside contacts of the EIS sensor chip were protected
mounted into the measuring cell; before testing, the sensors
from the electrolyte solution by means of an O-ring,
were preconditioned in phosphate buffer solution for at least
thereby circumventing the need for a complex encapsula-
2 h. Unless otherwise stated, the impedance measurements
tion process. The contact area of the EIS sensor with the
were carried out in 18 mM phosphate buffer, pH 7.4, in a
solution is determined by the diameter of the O-ring and
frequency range varying from 1 Hz up to 1 kHz. C–V
was about 0.5 cm2. All measurements were performed in a
measurements were carried out at 100 Hz frequency in the
dark Faraday cage at room temperature. All potential values
voltage range from −4 V to 1 V. The ConCap measurements
are referred to the reference electrode.
were performed at a frequency of 100 Hz, unless otherwise
The electrochemical characterization of the EIS sensors
was performed by means of capacitance–voltage (C–V),impedance spectroscopy, and constant-capacitance (ConCap)methods using an impedance analyzer (Zahner Elektrik,
Results and discussion
Kronach). For operation, a direct current polarisation voltagewas applied via the reference electrode to set the working
Sensor surface characterization
point of the EIS sensor, and a small alternating currentvoltage (20 mV) was applied to the system in order to
The fabrication of the sensor was a multistage process
measure the capacitance of the sensor. The sensor chips were
(Fig. involving silanization, gold colloid deposition,
Fig. 3 Electrochemical charac-
Initial sensor (1)
terization of the EIS sensor by
means of C–V measurements
Gold colloid modification (3)
after each preparation step. The
O2 plasma treatment (4)
inset shows the shift of
CD modification of gold surface (5)
flat-band voltage
FBP shift, mV -400
Voltage, V
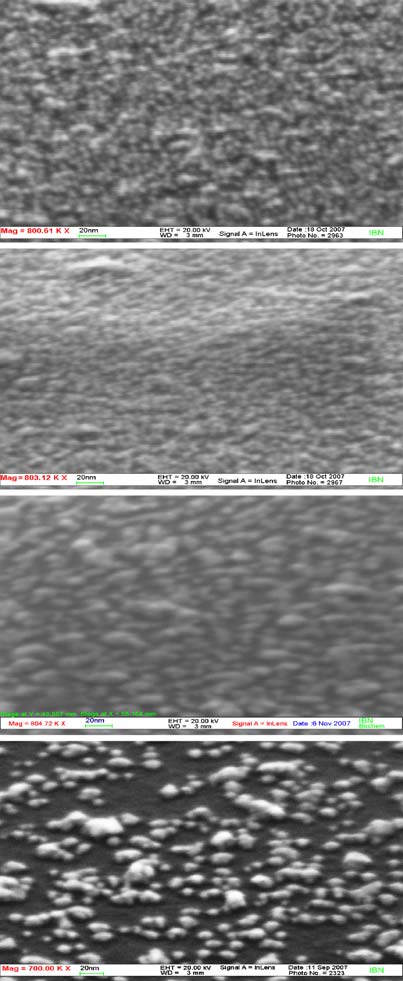
Oxygen plasma-treated gold nanoparticle-based field-effect devices
Table 1 Contact angle and flat-band potential shifts (FBP) by
different substrate treatments of the EIS structure
Preparation steps
Contact angle (°)
plasma treatment, and specific surface modification of thebare gold nanoparticles (NP). In the following, thenecessity of each preparation step and the correspondingflat-band potential shifts of the EIS sensor associated withthe different modifications will be briefly discussed.
Changes in the capacitive characteristics (C–V curves) andflat-band potential (FBP) shifts can be seen from Fig. and
are summarized in Table .
Notably, the silanization shifts the flat-band voltage by
some 30 mV (Table ) in the negative direction due to apartial coverage of surface silanols with gold nanoparticles.
Carrying out the silanization procedure in a less dryenvironment or for a prolonged duration resulted in up toten times thicker films (as observed by the ellipsometricmeasurements) and positive FBP shift, underscoring theeffect of the silanol groups in the silicate coatings. As
expected, the silanization step is also accompanied by asignificant surface hydrophobization, which is manifestedin an increase of the water wetting angle from 3° to 62°.
The subsequent addition of gold nanoparticles shifts thepotential back into positive direction. A possible explana-tion for this observed shift is the cumulative effect ofsurface silanols blocked by negatively charged NP. Thenegative charge of the gold NP was reported before, and itis attributed to the thiol–gold capping reaction , ].
Gold deposition further increases the contact angle, sincethe hydrophobic alkyl group is oriented outwards, towards
the solution, due to the ammonium group having a higheraffinity to the gold and thus being attached to the gold
Silanized surface
Gold modified
Our preliminary studies revealed that sensors fabricated
by surface modification of the capped gold NP suffered from
low sensitivity, low reproducibility and had a somewhat
sluggish behaviour. We attributed this malfunction to thepoor affinity of the tetraalkylammonium bromide to the gold
surface. Gradual loss of the charged moieties in aqueous
Fig. 4 a SEM images of gold NP-modified EIS sensor before and b
after oxygen plasma treatment of different power (time of the
treatment is 10 s). b Reflectance spectroscopy of initially silanizedand gold NP-modified EIS sensors as in a
J. Gun et al.
solution results in uncontrolled sensor drifts. Moreover, the
Table 2 XPS elemental analysis normalized to 100%
competition of the ammonium capping layer with the
Silanized surface
selective modifier, which is necessary for the specific sensing
element, results in a low surface coverage of the gold NP bythe selective modifier. Thus, we have two seemingly contra-
dicting effects: on the one hand, more potent capping agents
result in lower loading of the selective modifier, and on the
other hand, less effective capping agents with lower affinity
to the substrate are gradually lost upon prolonged immersion
in the test solutions. Our way to overcome this contradiction
is to take off the capping agent altogether.
Comparison of wet chemistry methods and dry "physically"
Relative error estimate =±15%a 200 W, 10 s
oriented methods clearly showed the advantage of oxygenplasma treatment to remove the organic moieties. We havecarried out fundamental research in order to optimize the
surface reduces the surface concentration of the Si and O
performance of the oxygen plasma clean-up process. The set of
elements and gives rise to new gold, nitrogen, and bromide
SEM micrographs of Fig. a summarizes visually the
signals. The latter are attributed to the gold NP capping agent
optimization criterion of the plasma treatment. Prior to the
tetraalkylammonium bromide. The sulphur content remained
application of the plasma treatment one observes a corrugated
approximately the same within the experimental error. The
surface densely packed by the gold NP. The density and
plasma treatment (200 W, 10 s) cleaned the surface of the
morphology of the nanoparticles remain essentially similar
sensor from the gold NP tetraoctylammonium bromide
even after exposure to 100 and 200 W plasma treatments.
capping agent, as manifested by the complete removal of
However, exposure of the films to 300 W and higher while
the bromide and nitrogen, though traces of sulphur were still
maintaining the same exposure duration (10 s) resulted in a
retained, as previously reported for plasma treated SAM of
distinctly lower coverage of the SiO2 surface by the gold NP.
alkyl thiolates on gold [].
Therefore, we applied 200 W plasma treatment for thesubsequent sensor fabrication. Figure provides UV–vis
Examples of sensing applications
reflectance measurements, which show that the plasmonpeak wavelength was indifferent to the power of the
In order to demonstrate the versatility and usefulness of this
plasma treatment, underscoring the fact that no nanoparticle
general approach we describe four different applications
coalescence/aggregation was imposed by the 200 W treat-
that are based on a gold NP-modified EIS platform.
ment. This observation commensurates with Boyen's report] that no change in the gold NP size histogram was
EIS-based pH sensor functionalized with gold NP
observed by oxygen plasma treatment, though Boyen'streatment was conducted with a lower density of gold
The basis for most enzymatic field-effect sensors con-
nanoparticle coverage and at lower power intensity (50 W).
structed with Si/SiO2 structures is its sensitivity (about 30–
The oxygen plasma removal of the hydrophobic capping
35 mV/decade , ]) to pH changes. Although higher
agents resulted in a lower wetting angle (61°), reflecting the
pH sensitivities were achieved by Ta2O5- or Si3N4-based
expected small loss of hydrophobicity. The sensor fabrica-
EIS sensors, SiO2-based EIS sensors remain an attractive
tion was then completed by covalent or physical linkage of
alternative for coupling biomolecules by means of sophis-
the desirable sensing moiety to the bare gold
ticated surface chemistry [–]. In the experiment,
To complete the description of sensor fabrication in
ConCap measurements (conducted at C=38 nF, 100 Hz)
Table , the modification of the gold NP-coated sensor by
in different commercial (titrisol, Merck) buffer solutions
aminomethyl-appended β-cyclodextrin moiety slightly
have been performed. Figure demonstrates the dynamic
increases the wetting angle (despite its hydrophilic character).
behaviour of an oxygen plasma-treated gold NP-based EIS
The first three steps of sensor preparation (silanization,
sensor. The average pH sensitivity of was about 33 mV/pH
gold modification, and plasma treatment with 200 W for 10 s)
in the pH range between pH 3 and pH 10. The charac-
were additionally accompanied by XPS analysis, and the
teristics of those EIS sensors were almost not influenced by
relevant observations are summarized in Table The
the gold deposition and plasma treatment when compared
determination of the elements was performed after subtrac-
to a bare Si/SiO2 sensor without gold NP. This indifference
tion of a Shirley background by fitting Gaussian–Lorentzian
in the pH sensitivity to the presence of gold NP can be
peaks to the region of interest measured with high energy
explained by the absence of any weak acid moieties on the
resolution. As it can be seen, gold coating of the silanized
gold surface. The fundamental sensing mechanism, i.e. the
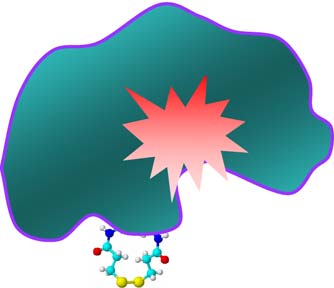
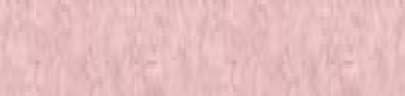
Oxygen plasma-treated gold nanoparticle-based field-effect devices
transducer surface of the sensor and allows a direct and fast
sensing of the reaction in case of enzyme-modified EIS
Despite the high popularity of nanoparticles supported
enzyme amperometric biosensors [these devices did
not find EIS applications yet.
Our first exemplifying test case is the conversion of
Voltage, V -1.40
glucose to gluconic acid by glucose oxidase. Since the pKa
of gluconic acid is 3.6 (K=2.5×10−4) at 20 °C ], this
reaction is accompanied by a change of the pH under near
neutral and basic buffers (see Eq.
2GLUCONATE þ H2O2 2Hþ:
Fig. 5 Typical ConCap measurement of a pH-sensitive EIS sensor
modified with gold NP in Titrisol buffer solutions from pH 3 to pH 10
The immobilization of the enzyme onto the gold surface
was done according to the schematic presentation in
acid-base titration of the surface silanol groups remains
Fig. The protocol starts with linkage of the dithio
nearly not-influenced by the partial coverage of gold NP.
groups of DSP on the gold surface, and then chemicalcross-linking of protein primary amine groups through
Gold NP-functionalized glucose EIS biosensor
succinimide group hydrolysis and formation of the amidebonds. This immobilization scheme was first proposed by
The pH sensitivity of bare EIS sensors is frequently used in
Katz for the derivatization of gold surfaces ] and proved
order to detect products of analytes that are converted by
to be useful for the gold NP modification as well.
enzymatic catalysis yielding pH changes EIS-based
In case of the NP-functionalized glucose EIS biosensor,
biosensors are especially suitable for sensing oxidoreductase-
the sensor stability and enzyme activity with and without
originated reactions, since the oxidation (or reduction)
the oxygen plasma treatment have been compared. The test
activity is often accompanied by an acidification of the
conditions followed the standard enzymatic assay of Sigma
analyte solution (pH change). This reaction is confined to
). The spectrophotometric de-
the immediate vicinity of the enzyme immobilized to the
tection of the enzymatic activity of the immobilized GOx in
Voltage, mV
GOx in the solution
Glucose concentration, mg/L
Fig. 6 a Typical glucose calibration curve for a gold NP-modified EIS sensor with surface-immobilized enzyme and in a GOx solution (buffer:PBS 4.5 mM, pH 7.5). b Reaction scheme of the gold NP-functionalized glucose EIS biosensor
J. Gun et al.
the buffer solution was done by tracing the development of
the colour (A500) by the oxidation of o-dianisidine. This dye
was catalytically oxidized by hydrogen peroxide in the
presence of HRP. To conduct the assay with a solid support,
the EIS sensor (0.9×0.9 cm) was placed on the bottom of a
3.1 ml spectrophotometric cell with a light path of 1 cm.
Voltage, mV -0.75
Assay concentrations were 48 mM sodium acetate,0.16 mM o-dianisidine, 1.161% (w/v) glucose, and 6 U
HRP (EC 1.11.1.7) [Table compares the investigated
assays obtained for the EIS sensors before and after plasma
treatment. The plasma-treated GOx sensor exhibited abetter initial response, and its storage stability at 4 °C
(PBS, 4.5 mM buffer, pH 7.5) was much superior to the
80 mV/decade
sensor that did not undergo the oxygen plasma treatment.
Figure depicts two typical calibration curves achieved
from ConCap measurements for a GOx-modified gold NP
63 mV/decade
EIS sensor that is compared with a blank gold NP EIS
20 mV/decade
sensor (unmodified with GOx), where for the latter the
enzymatic reaction was conducted by adding the GOx to
Voltage change, mV
13 mV/decade
the solution. In this experiment, the solution contained 12 U
of GOx per ml, whereas on the GOx-immobilized sensor
Penicillin concentration, mM
chip there were 4.0 U of glucose oxidase (determined by
Fig. 7 a Typical ConCap measurements of penicillin concentration
the spectrophotometric assay in Table Both calibration
with gold NP-modified EIS sensor with chemically coupled and
plots follow the Michaelis–Menten dependency with
physically adsorbed penicillinase, and b corresponding calibration
curves in different ranges of penicillin concentration
m values. However, the enzyme-modified EIS
glucose biosensor reacts faster and exhibits a much superiorsensitivity compared to the blank EIS sensor that "only
pH-sensitive substrates [In this study, we compared
works" as a pH sensor.
two modes of penicillinase modification of gold NP-functionalized EIS sensors, chemical coupling by DSP
Gold NP-functionalized penicillin EIS biosensor
reagent and physical adsorption. As can be seen in Fig. both biosensors are sensitive to a wide range of penicillin
The enzyme penicillinase was introduced for the first time
concentrations (from 0.25 to 10 mM). Nonetheless, the
for constructing a potentiometric penicillin biosensor by
sensitivity of the chemically coupled penicillinase biosensor
Shearer ] and for field-effect devices by Janata [Due
is distinctly higher as in the case of biosensor that was
to the high importance of penicillin determination for
modified by physisorption, though both operate under
biomedical measurements, the development of improved
identical conditions (see also Fig.
penicillin sensors is still a contemporary challenge [].
Penicillinase converts penicillin to penicilloic acid, which
releases H+ ions ] at moderate and basic pH. The pH
change is determined by the EIS sensor. Penicillinase is ahighly adhesive enzyme, and penicillin sensors can be
prepared by simple adsorptive immobilization on different
Pb2+ 10 -7 M
Table 3 Enzymatic activity (U/sensor) as a function of storage time
Pb2+ 10-6 M
and plasma treatment for the gold NP-structured EIS biosensor
Voltage, V
Pb2+ 10-5 M
Pb2+ 10-4 M
Time after sensor
GOx on gold NP-modified GOx on gold NP-
preparation (days) sensor after plasma
Pb2+ 10-3 M
treatment (U/sensor)
treatment (U/sensor)
Fig. 8 Typical ConCap response of a gold NP-functionalized EIS
sensor modified with aminomethyl β-cyclodextrine to sequentialaddition of lead nitrate in 18 mM PBS, pH 7.2
Oxygen plasma-treated gold nanoparticle-based field-effect devices
β-cyclodextrin-modified gold NP-functionalized EIS sensor
different methods and examples for the attachment of an
for lead ion detection
ionophore and two enzymatic sensing elements, respec-tively: Physisorption for penicillinase, DSP anchoring for
As an example for a gold NP-functionalized chemical EIS
glucose oxidase and penicillinase, and amine linkage for β-
sensor, we demonstrate here a β-cyclodextrin-modified lead
CD. Moreover, functionalized gold nanoparticles are highly
ion sensor. β-cyclodextrin (β-CD) is a cyclic oligosaccharide
compatible with Si chip technology and provide exceptional
comprised of seven glucopyranose units Cyclodextrins
versatility in chip functionalization and field-effect sensor
are well known agents for including complexes formation
]. A large assortment of guest molecules, from simpleions to complicated organic molecules of ionic or neutral
The authors are grateful for the technical
assistance of H.-P. Bochem and A. Besmehn for the surface
nature, undergoes the inclusion ligation by different CDs
characterization with HRSEM and XPS and to A. Voskevich for the
[]. Since CDs are water-soluble, the use of these
very useful discussions. J. Gun thanks the Alexander von Humboldt
molecules as ionophores for sensor fabrication has to
Foundation for the financial support.
involve some sort of immobilization. For this purpose, inprevious reports CDs were incorporated in a PVC gelmembrane with polymethylhydrosiloxane ] or were
copolymerized with a polysiloxane gel ]. However,generally membranes lower the response time of sensors
1. Brust M, Walker M, Bethell D, Schiffrin DJ, Whyman R (1994)
Synthesis of thiol-derivatised gold nanoparticles in a 2-phase
and might negatively affect their sensitivity. In this
liquid–liquid system. J Chem Soc Chem Commun 7:801–802
experiment, we chose to attempt a direct surface modifica-
2. Guoa S, Wang E (2007) Synthesis and electrochemical applications
tion of gold NP with host ionophore molecules as an
of gold nanoparticles. Anal Chim Acta 598:181–192
alternative approach for CD sensor fabrication. The sensor
3. Kharitonov AB, Shipway AN, Katz E, Willner I (1999) Gold-
was constructed by the procedure described in the experi-
sensitive field-effect transistors: novel assemblies for the sensing
mental section. To elucidate the influence of specific
of neurotransmitters. Anal Chem 18:255–260
modification of the gold NP-functionalized sensor with the
4. Katz E, Willner I (2003) Probing biomolecular interactions at
ionophore on the ion caption process, we compared the
conductive and semiconductive surfaces by impedance spectros-copy: routes to impedimetric immunosensors, DNA-sensors, and
response of the plasma-treated gold NP sensor before and
enzyme biosensors. Electroanalysis 15:913–947
after its modification with aminomodified CD.
5. Kielbassa S, Habich A, Schnaidt J, Bansmann J, Weigl F, Boyen
Figure describes the dynamic response of the EIS
HG, Ziemann P, Behm RJ (2006) On the morphology and stability
sensor to sequential addition of lead nitrate. All measure-
of Au nanoparticles on TiO2(110) prepared from micelle-stabilizedprecursors. Langmuir 22:7873–7880
ments were done in 18 mM PBS solution, pH 7.2. At the
6. Raiber K, Terfort A, Benndorf C, Krings N, Strehblow HH (2005)
low concentration range (below 10−5 M) the response was
Removal of self-assembled monolayers of alkanethiolates on gold
rather fast and it took less than 40 s to stabilize the sensor
by plasma cleaning. Surf Sci 595:56–63
signal. The calibration curve (not shown) was linear in the
7. Hesse E, Creighton JA (1999) Investigation by surface-enhanced
Raman spectroscopy of the effect of oxygen and hydrogen
concentration range 10−7 to 10−3 M with a slope of 8±
plasmas on adsorbate-covered gold and silver island films.
0.6 mV/decade. In comparison, a blank, oxygen plasma-
Langmuir 15:3545–3550
treated gold NP–EIS sensor shows less than 1 mV per
8. Gun J, Schöning MJ, Abouzar MH, Poghossian A, Katz E (2008)
decade signal changes, which can be attributed to a general
Field-effect nanoparticle-based glucose sensor on a chip: ampli-fication effect of co-immobilized redox species. Electroanalysis
drift of the sensor rather than to a response towards lead
ions. The sensitivity of the developed lead sensor is
9. Brust M, Bethell D, Schiffrin DJ, Kiely CJ (1995) Novel gold-
somewhat lower compared to prior EIS lead sensors based
dithiol nano-networks with nonmetallic electronic-properties. Adv
on β-cyclodextrin but the linear range and the
Mater 7:795–803
10. Fishelson N, Shkrob I, Lev O, Gun J, Modestov AD (2001) Studies
minimum detection level are much more improved com-
on charge transport in self-assembled gold dithiol films: conduc-
pared to prior reports ].
tivity, photoconductivity and Photoelectrochemical measurements.
Langmuir 17:403–412
11. Schmitt J, Machtle P, Eck D, Mohwald H (1999) Preparation and
optical properties of colloidal gold monolayers. Langmuir
12. Russell CP, Salek JS, Sikorski CT, Kumaravel G, Lin FT (1990)
The preparation of gold NP-modified silicon-based EIS
Cooperative binding by aggregated mono-6-(alky1amino)-β-
sensors was described with an emphasis on the importance of
cyclodextrins. J Am Chem Soc 112:3860–3868
13. Thermo Scientific (2007) Tech Tip #2: attach a protein onto a gold
oxygen plasma treatment after the gold NP-functionalization
surface. Available at
with regard to the sensor sensitivity and stability. The
14. Tang DY, Xia BY, Zhang YQ (2008) Direct electrochemistry
versatility of the gold NP platform is demonstrated by three
and electrocatalysis of hemoglobin in a multilayer {nanogold/
J. Gun et al.
PDDA}(n) inorganic-organic hybrid film. Microchim Acta 160:
31. Parke SA, Birch GG, MacDougall DB, Stevens DA (1997) Tastes,
structure and solution properties of D-glucono-1,5-lactone. Chem
15. Wuelfing WP, Green SJ, Pietron JJ, Cliffel DE, Murray RW (2000)
Senses 22:53–65
Electronic Conductivity of solid-state, mixed-valent, monolayer-
32. Katz EY (1990) A chemically modified electrode capable of a
protected Au clusters. J Am Chem Soc 122:11465–11472
spontaneous immobilization of amino-compounds due to its
16. Poghossian A, Abouzar MH, Sakkari M, Kassab T, Han Y,
functionalization with succinimidyl groups. J Electroanal Chem
Ingebrandt S, Offenhäusser A, Schöning MJ (2006) Field-effect
sensors for monitoring the layer by-layer adsorption of charged
33. Bergmeyer HU, Gawehn K, Grassl M (1974) In: Bergmeyer HU
macromolecules. Sens Actuators B Chem 118:163–170
(ed) Methods of enzymatic analysis, vol 1, 2nd edn. Academic,
17. Cane C, Gracia I, Merlos A (1997) Microtechnologies for pH
NY, USA, pp 457–458
ISFET chemical sensors. Microelectron J 28:389–405
34. Papariello GJ, Mukherji AK, Shearer CM (1973) Penicillin
18. Schöning MJ, Poghossian A (2006) BioFEDs (Field-effect devices):
selective enzyme electrode. Anal Chem 45:790–792
state-of-the-art and new directions. Electroanalysis 18:1893–1900
35. Caras S, Janata J (1980) Field-effect transistor sensitive to
19. Schöning MJ, Tsarouchas D, Schaub A, Beckers L, Zander W,
penicillin. Anal Chem 52:1935–1937
Schubert J, Kordos P, Lüth H (1996) A highly long-term stable
36. Poghossian A, Thust M, Schroth P, Steffen A, Lüth H, Schöning
silicon-based pH sensor using pulsed laser deposition technique.
MJ (2001) Penicillin detectionby means of silicon-based field-
Sens Actuators B Chem 35:228–233
effect structures. Sens Mater 13:207–223
20. Poghossian A, Schöning MJ (2007) In: Grimes CA, Dickey EC,
37. Poghossian A, Yoshinobu T, Simonis A, Ecken H, Lüth H,
Pishko MV (eds) Encyclopedia of sensors, chapter 24, vol 9.
Schöning MJ (2001) Penicillin detection by means of field-effect
American Scientific, Stevenson Ranch, USA, pp 463–534
based sensors: EnFET, capacitive EIS sensor or LAPS? Sens
21. Poghossian A, Schöning MJ (2008) In: Marks RS, Cullen DC,
Actuators B Chem 78:237–242
Karube I, Lowe CR, Weetall HH (eds) Handbook of biosensors
38. Poghossian A, Schöning MJ, Schroth P, Simonis A, Lüth H
and biochips, chapter 24. Wiley, Weinheim, Germany, pp 1–17
(2001) An ISFET-based penicillin sensor with high sensitivity,
22. Schöning MJ, Brinkmann D, Rolka D, Demuth C, Poghossian A
low detection limit and long lifetime. Sens Actuators B Chem
(2005) CIP (cleaning-in-place) suitable "non-glass" pH sensor
based on a Ta2O5-gate EIS structure. Sens Actuators B Chem
39. Poghossian A, Thust M, Schöning MJ, Müller-Veggian M,
Kordos P, Lüth H (2000) Cross-sensitivity of a capacitive penicillin
23. Thust M, Schöning MJ, Schroth P, Malkoc Ü, Dicker CI, Steffen A,
sensor combined with a diffusion barrier. Sens Actuators B Chem
Kordos P, Lüth H (1999) Enzyme immobilisation on planar and
porous silicon substrates for biosensor applications. J Mol Catal B
40. Wang J (1988) Electroanalytical techniques in clinical chemistry
and laboratory medicine. Wiley-VCH, Weinheim, Germany
24. Liao CW, Chou JC, Sun TP, Hsiung SK, Hsieh JH (2007)
41. Thust M, Schöning MJ, Vetter J, Kordos P, Lüth H (1996) A long-
Preliminary investigations on a glucose biosensor based on the
term stable penicillin-sensitive potentiometric biosensor with
potentiometric principle. Sens Actuators B Chem 21:720–726
enzyme immobilized by heterobifunctional crosslinking. Anal
25. Yao K, Zhu YH, Wang P, Yang XL, Cheng PZ, Lu H (2007)
Chim Acta 323:115–121
ENFET glucose biosensor produced with mesoporous silica
42. Szejtli J (1988) Cyclodextrine technology. Kluwer, Boston,
microspheres. Mater Sci Eng C 27:736–740
26. Xiao Y, Patolsky F, Katz E, Hainfeld JF, Willner I (2003)
43. Li S, Purdy WC (1992) Cyclodextrins and their applications in
"Plugging into enzymes": nanowiring of redox enzymes by a
analytical-chemistry. Chem Rev 92:1457–1470
gold nanoparticle. Science 299:1877–1881
44. Lahiani-Skiba M, Coquard A, Bounoure F, Verite P, Arnaud P,
27. Cavaliere-Jaricot S, Darbandi M, Kucur E, Nann T (2008) Silica
Skiba M (2007) Mebendazole complexes with various cyclo-
coated quantum dots: a new tool for electrochemical and optical
dextrins: preparation and physicochemical characterization. J Incl
glucose detection. Mikrochim Acta 160:375–383
Phenom Macrocycl Chem 57:197–201
28. Bharathi S, Lev O (1998) Sol-gel-derived nanocrystalline gold-
45. Ben Ali M, Kalfat R, Sfihi H, Ben Ouada H, Chovelon JM,
silicate composite biosensor. Anal Commun 35:29–31
Jafferezic-Renault N (1998) Cyclodextrin-polymethylhydrosilox-
29. Liu Y, Hu LM, Yang SQ (2008) Amplification of bioelectrocatalytic
ane gel as sensitive membrane for heavy ion sensors. Mater Sci
signalling based on silver nanoparticles and DNA-derived horserad-
ish peroxidase biosensors. Mikrochim Acta 160:357
46. Ben Ali M, Kalfat R, Sfihi H, Ben Ouada H, Chovelon JM,
30. Zhao J, Yu JJ, Wang F, Hu SS (2006) Fabrication of gold
Jafferezic-Renault N (2000) Sensitive cyclodextrin-polysiloxane
nanoparticle-dihexadecyl hydrogen phosphate film on a glassy
gel membrane on EIS structure and ISFET for heavy metal ion
carbon electrode. Mikrochim Acta 156:277–282
detection. Sens Actuators B Chem 62:233–237
Source: http://team.fh-kl.de/fileadmin/team/maryam-weil/G5.pdf
Literature Review on Men, Gender, Health and HIV and AIDS in South AfricaAugust 2008Dean Peacock, Jean Redpath, Mark Weston, Kieran Evans, Andrew Daub and Alan Greig for Sonke Gender Justice Network. Sable Centre, 16th Floor 41 De Korte Street Braamfontein 2017 T: +27 11 339 3589 F: +27 11 339 6503 Cape Town Office:
OFFICE OF PUBLIC WORKS Before the Deluge Tony Smyth, Director of Engineering Services and Chief Engineer in the Office of Public Works, talks John Walshe through the OPW's efforts to revolutionise flood risk management in Ireland via the Catchment Flood Risk Assessment and Management (CFRAM) programme. The CFRAM programme is set EU FLOODS DIRECTIvE