Mechanical competence of ovariectomy-induced compromised bone after single or combined treatment with high-frequency loading and bisphosphonates
OPEN Mechanical competence of
ovariectomy-induced compromised
bone after single or combined
Received: 10 February 2015
Accepted: 28 April 2015
treatment with high-frequency
Published: 01 June 2015
loading and bisphosphonates
Camargos G. V.1,3, Bhattacharya P.2, van Lenthe G. H.2, Del Bel Cury A. A.3, Naert I.1, Duyck
J.1 & Vandamme K.1
Osteoporosis leads to increased bone fragility, thus effective approaches enhancing bone strength
are needed. Hence, this study investigated the effect of single or combined application of high-
frequency (HF) loading through whole body vibration (WBV) and alendronate (ALN) on the
mechanical competence of ovariectomy-induced osteoporotic bone. Thirty-four female Wistar rats
were ovariectomized (OVX) or sham-operated (shOVX) and divided into five groups: shOVX, OVX-
shWBV, OVX-WBV, ALN-shWBV and ALN-WBV. (Sham)WBV loading was applied for 10 min/day
(130 to 150 Hz at 0.3g) for 14 days and ALN at 2 mg/kg/dose was administered 3x/week. Finite
element analysis based on micro-CT was employed to assess bone biomechanical properties,
relative to bone micro-structural parameters. HF loading application to OVX resulted in an enlarged
cortex, but it was not able to improve the biomechanical properties. ALN prevented trabecular bone
deterioration and increased bone stiffness and bone strength of OVX bone. Finally, the combination
of ALN with HF resulted in an increased cortical thickness in OVX rats when compared to single
treatments. Compared to HF loading, ALN treatment is preferred for improving the compromised
mechanical competence of OVX bone. In addition, the association of ALN with HF loading results in
an additive effect on the cortical thickness.
Osteoporosis is a skeletal disease characterized by low bone mass and micro-architectural deterioration
of the bone tissue, leading to decreased bone strength predisposing increased risk of fractures. This dis-
ease is associated with estrogen deficiency after menopause, which is responsible for shifting the balance
between bone resorption and bone formation toward an increased level of bone resorptio. With an
increasing aging population worldwide, osteoporosis has become a growing health concern, since the
augmented risk of bone fractures has devastating outcomes in terms of mortality, decreased autonomy
and healthcare costs. Thus, therapies that increase the bone mechanical competence in medical com-
promised conditions are required.
The current therapies to prevent bone loss in estrogen-deficient state are via drugs that affect bone
metabolism, such as biphosphonates (BPs). The biphosphonate drug alendronate is a potent anti-resorptive
ageith evidenced reduction of fracture risk in postmenopausal womowever, it has some
1Department of Oral Health Sciences, BIOMAT Research Cluster & Prosthetic Dentistry, KU Leuven & University Hospitals Leuven, Leuven, Belgium. 2Biomechanics Section, Department of Mechanical Engineering, KU Leuven, Leuven, Belgium. 3Department of Prosthodontics and Periodontology, Piracicaba Dental School, University of Campinas, Piracicaba, São Paulo, Brazil. Correspondence and requests for materials should be addressed to V.K. (email: )
Scientific
RepoRts 5:10795 DOi: 10.1038/srep10795
limitations and side effects that affect long-term administration and patient's adheren. Alternatively
to the pharmacological treatment with BPs, biomechanical therapies have been proposed to treat osteo-
porosis due the profound anabolic effects of mechanical loading on bonIn particular, the application
of high-frequency (HF) loading via whole body vibration (WBV) has been shown to promote bone
formation, and even recover bone loss arising from osteoporotic conditions
The anti-fracture efficacy of these osteoporosis therapies was assessed in previous studies by using dual
energy X-ray absorptiometry (DXA) based on bone mineral density . However, DXA-BMD is
not an appropriate predictor for the strength of the bone because of the poor association between BMD
change and bone fragilitys the method is based on a two-dimensional projection measurement of the
three-dimensional bone structure, it lacks the ability to explore macro- and micro-architectural features
of the bon. Thus, fracture risk cannot be directly inferred from BMD, and surrogates for assessment
of bone mechanical competence that consider bone geometric aspects are needed.
In this context, finite element (FE) analysis provides an approach to assess bone biomechanical
properties, particularly when combined with specimen-specific high-resolution data obtained from
micro-computed tomography, from which the bone micro-architecture can be easily obtaine. Therefore,
the aims of the present study were: (i) to investigate the effect of single or combined application of HF
WBV and ALN on the mechanical competence of bone originating from osteoporotic animals; and (ii)
to determine how the changes in trabecular and cortical bone micro-structure after these interventions
contribute to the bone biomechanical properties.
Animals and experimental design. The protocol of the animal experiment was approved by the
local ethical committee (P050/2011), complied with ARRIVE guidelin for preclinical studies and was
performed according to the Belgian animal welfare regulations and guidelines.
A total of 34 female Wistar rats at 12 weeks of age were used in the present study. Twenty-seven
animals underwent ovariectomy surgery [OVX], while the remaining seven animals were subjected
to sham-ovariectomy surgery [shOVX]. (Sham)-ovariectomy surgery was performed at Charles River
Laboratories (Charles River, L' Arbresle, France). For the rats subjected to sham surgery, the bilateral
ovaries were lifted up and returned to their original position, while for the ovariectomized rats, the ova-
ries were removed. Rats arrived 5 days post-(sham)OVX surgery, with a body weight ranging between
220 g and 250 g. Pair-feeding regimen was initiated immediately in an attempt to control the body weight
changes throughout the study. The average daily food consumption of the shOVX animals was deter-
mined, and the quantified amount was then provided to the other animals. Animals were weighed at
the start and once a week during the study. The OVX group was divided into 2 groups, an untreated
group [OVX] (n = 13) and a group treated with the anti-resorptive bisphosphonate drug alendronate
[ALN] (n = 14). Alendronate sodium trihydrate (A4978-100MG, Sigma-Aldrich, Bornem, Belgium) was
injected subcutaneously 3 days/week at a dose of 2 mg/kg body weight/dose, starting 5 days post-OVX
surger. Saline administration (0.9% NaCl) was performed according to the same time schedule to the
rats of the OVX and shOVX groups. Injections were administered till the day of euthanasia (i.e. 14 days
after starting the mechanical stimulation). The animals of OVX and ALN groups were further divided
into subgroups relative to the loading condition (sham-WBV
versus WBV), resulting in 4 experimental
groups (OVX-shWBV, n = 7; OVX-WBV, n = 6; ALN-shWBV, n = 6; ALN-WBV, n = 8). The 5th group,
namely the shOVX group (n = 7), did not receive mechanical stimulation, and served as a negative con-
trol to il ustrate normal bone characteristics over the course of the experiment. The OVX-shWBV group
served as positive control to demonstrate the changes occuring during the development of osteoporotic
condition following ovariectomy.
Vibration device and loading protocol. HF mechanical loading was initiated 6 weeks post-(sham)
ovariectomy. This time lapse was considered to be adequate for inducing significant bone changes
in the rat long bones in response to ovariectom. The loading was applied by means of WBV via a
custom-made vibration device. The WBV loading was administered during 14 days for 10 minutes/day
according to a protocol that consisted of 10 consecutive frequency steps (130, 135, 140, 145, 150, 130,
135, 140, 145 and 150 Hz), each of these applied for 1 minute at an acceleration of 0.3. WBV was
applied to the animals individual y, taking into account the animal's body weight. Intervals of 24-hours
between the loading sessions were respected.
Specimen preparation and micro-X-ray computed tomography analysis. After euthanasia of
the animals by cervical displacement under isoflurane-induced anaesthesia, the hindlimbs were excised
and the surrounding soft tissues removed. The tibiae were retrieved and immediately fixated in 10%
CaCO3–buffered formalin solution (pH 7.4) at 4 °C for 48 h. The samples were further kept in the 70%
ethanol at 4 °C until the day of μ CT scanning.
For assessment of the bone micro-architecture in the experimental groups, the proximal part of the
tibiae was examined
ex vivo using a desktop μ CT system, commercial y available as Skyscan 1172 (Bruker,
Kontich, Belgium). During scanning, the tibia was placed in the polyethylene tube and immobilized
inside the tubes by means of soft modeling clay. The bone samples were scanned along the mid-sagittal
Scientific
RepoRts 5:10795 DOi: 10.1038/srep10795
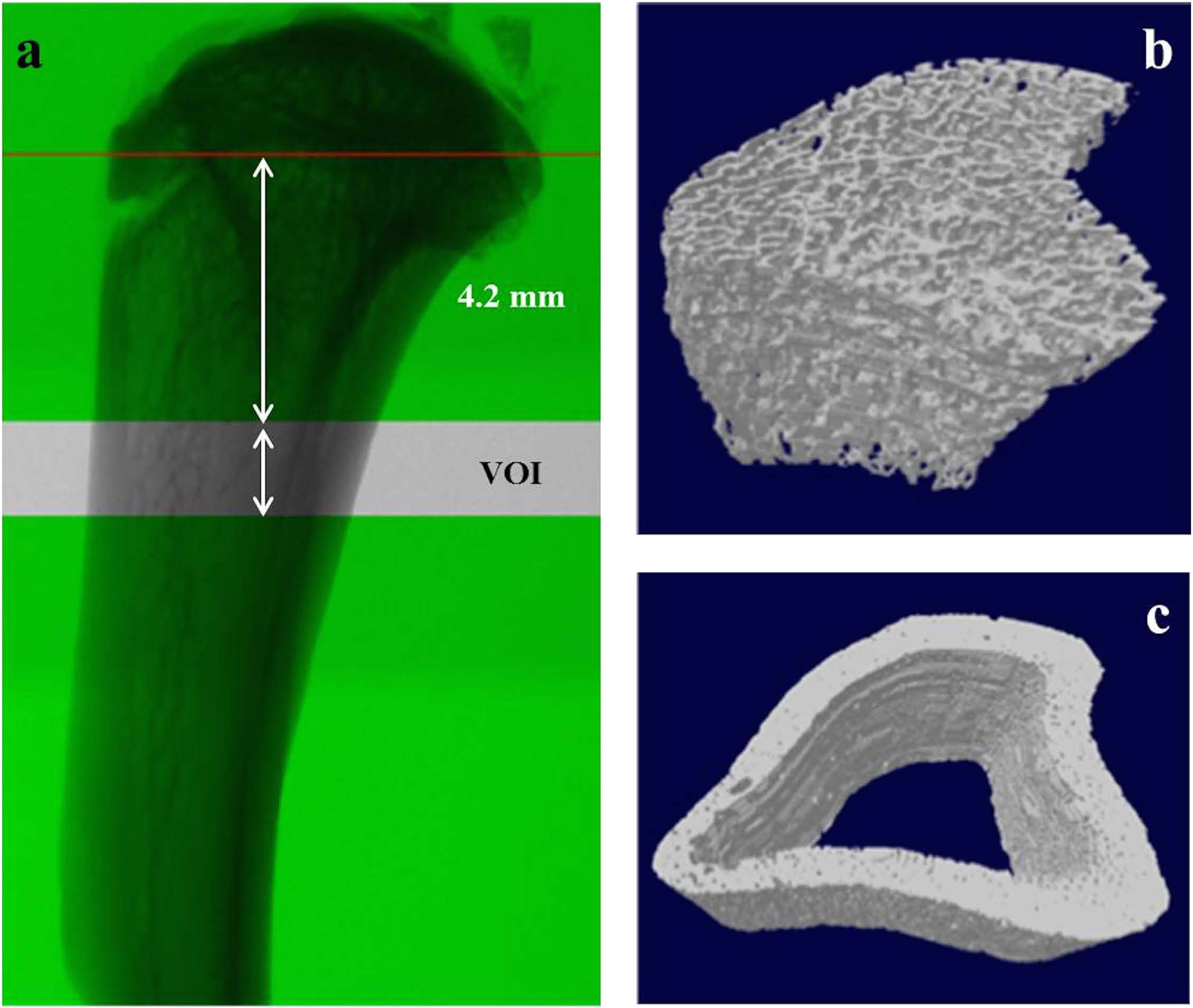
Figure 1. Representative μ CT images of rat tibia. (a) VOI selected for trabecular and cortical bone analysis:
4.2 mm distal y from the growth plate level and extending towards the distal diaphysis over a distance of
1.5 mm; (b) 3D view of trabecular (b) and cortical bone (c) volume reconstructed from the selected ROI.
planes in the mid-diaphyseal regions to obtain the μ CT images. The scanning parameters were 6 μ m pixel
size, 50 kV X-ray voltage, 200 μ A electric current and 0.5 mm Al filter.
Subsequently, the reconstructed 3D data sets were quantified using CTAn automated image analysis
system (Bruker, Kontich, Belgium). For this, the volume of interest (VOI) for both trabecular and cor-
tical analyses was defined in axial direction by using the growth plate as reference. The VOI started at a
distance of 4.2 mm distal y from the growth plate and extended towards the diaphysis for 1.5 mm (250
sn each transverse slice of the VOI, the ROI was delineated manual y matching with the
area occupied by trabecular cortical bone o perform trabecular or cortical bone
For trabecular bone analysis, the bone volume (BV), bone volume fraction (BV/TV), trabecular
thickness (Tb.Th), trabecular separation (Tb.Sp) and trabecular number (Tb.N) were calculated 3D as
measurements of trabecular bone mass and its distribution. The trabecular architecture was quantified
by calculating the connectivity of the trabecular network (trabecular bone pattern formation, TBPf) and
structure model index (SMI). For cortical bone analysis, the periosteal perimeter (Ps.Pm), medul ary area
(Ma.Ar), endocortical perimeter (Ec.Pm) and mean polar moment of inertia (MMI) were calculated in
2D as measurements of cortical bone mass and its distribution. The porosity (Ct.Po) and cortical thick-
ness (Ct.Th) were calculated 3D as measurements of cortical bone mass and its distribution according
to standard procedur.
Structural analysis. Finite element models of all bone samples were created by a direct conversion
of bone voxels to cubic hexahedral elemen. The three-dimensional (3D) models of bone presented a
fixed length in z-direction (3.0 mm) that corresponded to the VOI evaluated in the μ CT analysi
These models consisted of 3.8 to 6.4 million elements, having between 12.2 and 21.7 million degrees of
freedom. Linear and isotropic material behavior was assumed. Material properties were applied, using
typical values for bone (Young's modulus, E = 10 GPa and Poisson's ratio, ν = 0.3). Identical material
properties were used for all groups, thereby enabling to isolate the effects of micro-architectural differ-
ences on bone stiffness and bone strength. Boundary conditions that represented uniaxial compression
tests were defined in order to determine the bone mechanical competence. The nodes at the top surface
of samples were displaced in axial direction by 1% of total height. Since all models had the same z-extent,
1% of strain in z-direction corresponds to a standard z-displacement of 0.03 mm. All nodes at the bottom
Scientific RepoRts 5:10795 DOi: 10.1038/srep10795
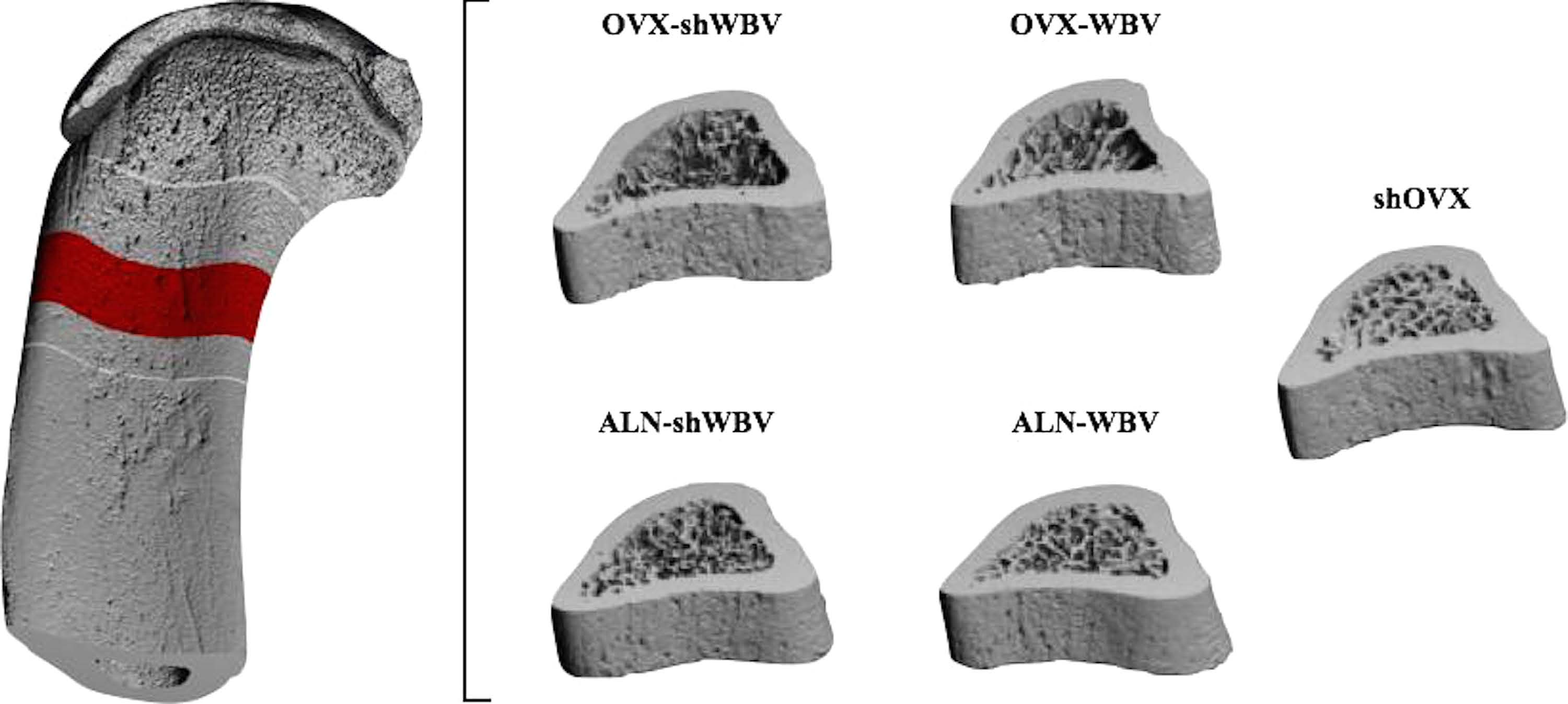
Figure 2. Representative three-dimensional models of the tibial diaphysis for each group (shOVX, OVX-
shWBV, OVX-WBV, ALN-shWBV and ALN-WBV) used in μ FE analysis.
of the models were restrained in the direction of the loading, except for two nodes that were restricted
in the transversal plane too, in order to prevent rigid body rotation and translations.
For the solution of these models with up to 20 million degrees of freedom, a parallel linear finite
element package (ParFE) with an algebraic multigrid preconditioner was use. All the calculations
were performed at the National Supercomputing Center (CSCS, ETH Zürich, Switzerland), where the
code was run on up to 3072 cores of a CRAY XT5™. The latter device consists of 3688 AMD hexa-core
Opteron processors clocked at 2.4 GHz and a high-speed interconnect with a bandwidth of 9.6 GB/s
and a latency of 5 μ s. Numerical post-processing was done on a Sun Fire™ system; for visualization, the
parallel open source program ParaVie was used, running on an HP-XC cluster. Bone stiffness [N/mm]
was determined from the FE model as the slope of the linear force–displacement relationship obtained
up to 1% apparent compressive strain. Bone strength was determined as the minimum force required
for 2% of the voxels (representing 2% of total bone volume) reaching at least 0.7% effective stra.
Statistical analysis. Results of the bone micro-structural and biomechanical parameters were
expressed as means ± standard deviation (SD). The differences between the means for the experimental
groups were compared using one-way analysis of variance (ANOVA). Multiple comparisons between
the groups were performed using Scott–Knott method and differences were considered significant at
p < 0.05.
Rats body weight. The body weight recordings at the day of arrival of the animals (i.e. 5 days post-
(sh)OVX surgery) revealed a slightly higher body weight for OVX and ALN animals compared to shOVX
animals (246.2 ± 14.6 g for OVX, 241.1 ± 13.9 g for ALN and 230.9 ± 14.1 g for shOVX). As a conse-
quence of ovariectomy surgery, the animals from OVX and ALN group indeed have the tendency to
gain weight compared to shOVX group, despite of the pair-feeding regime (272.5 ± 17.7 g for OVX,
268.7 ± 18.9 g for ALN and 233.0 ± 12.8 g for shOVX). However, HF WBV application did not affect the
rats' body weight changes in all experimental groups.
ANOVA results. ANOVA revealed a statistical y significant difference between the groups (shOVX,
OVX-shWBV, OVX-WBV, ALN-shWBV, ALN-WBV) for bone stiffness (p = 0.000), bone strength
(p = 0.000), trabecular micro-structural parameters (BV, p = 0.000; BV/TV, p = 0.000; Tb.Th, p = 0.014;
Tb.SP, p = 0.000; Tb.N, p = 0.000; TBPf, p = 0.000; SMI, p = 0.000) and cortical micro-structural param-
eters (Ct.Th, p = 0.001; Ct.Po, p = 0.011; Ps.Pm, p = 0.004; Ma.Ar, p = 0.012; and MMI, p = 0.001), except
for Ec.Pm (p = 0.056). The results of the multiple comparions between all experimental groups for each
biomechanical and micro-structural parameter are shown
Effect of WBV and/or ALN on bone micro-structure. OVX caused a marked deterioration of the
trabecular and cortical bone micro-structure, manifested as a considerable decrease in Ct.Th (− 9%),
BV (− 52.6%), BV/TV (− 63.6%), Tb.N (− 75.0%) and increase in Ma.Ar (+ 21.9%), Tb.Sp (+ 163.4%),
TBPf (+ 466.7%) and SMI (+ 144.4%) (OVX-shWBV versus shOnimultaneously, as
Scientific RepoRts 5:10795 DOi: 10.1038/srep10795
Mean ± Standard Deviation
Strength (N)
BV (μ m3)
BV/TV (%)
Tb.Th (μ m)
Tb.Sp (μ m)
Tb.N (1/mm) TBPf (1/mm)
68280.0 ± 1571.6b 1042.6 ± 32.1b
1.9E+ 09 ± 2.2E+ 08b
27.5 ± 2.5b
189.6 ± 8.0b
3.0 ± 3.0b
0.9 ± 0.3b
60974.0 ± 3311.8a
934.2 ± 59.0a
9.0E+ 08 ± 3.1E+ 08a
10.0 ± 4.7a
499.4 ± 231.3a
1.0 ± 1.0a
17.0 ± 4.0a
2.2 ± 0.3a
58399.9 ± 3989.2a
900.1 ± 63.5a
5.3E+ 08 ± 2.2E+ 08a
7.8 ± 3.1a
636.6 ± 250.4a
1.0 ± 1.0a
21.0 ± 4.0a+b
2.6 ± 0.3a
70596.1 ± 5729.3b 1063.2 ± 79.8b
1.9E+ 09 ± 4.5E+ 08b
22.0 ± 3.7a+b
57.7 ± 2.3a+b
208.7 ± 22.6b
4.0 ± 1.0b
6.0 ± 5.0b
1.1 ± 0.5b
69834.2 ± 4804.4b 1098.8 ± 69.8b
1.8E+ 09 ± 4.3E+ 08b
23.5 ± 3.3a+b
60.8 ± 6.3a+b
210.1 ± 13.9b
4.0 ± 1.0b
1.0 ± 0.2b
Table 1. Results for bone stiffness, bone strength and trabecular bone micro-structural parameters (BV, BV/
TV, Tb.Th, Tb.Sp, Tb.N, TBPf, SMI) for each group evaluated. Means ± SD followed by "a" and "b" represent
statistical y significant difference from the ShOVX and OVX-shWBV group, respectively (p < 0.05).
Mean ± Standard Deviation
Strength (N)
Ct.Po (%)
Ps.Pm (μ m)
Ma.Ar (μ m2)
MMI (μ m4)
Ec.Pm (μ m)
Ct.Th (μ m)
68280.0 ± 1571.6b
1042.6 ± 32.1b
13557.6 ± 399.3b
4.8E+ 06 ± 3.7E+ 05b
1.2E+ 13 ± 1.4E+ 12
451.6 ± 20.0b
60974.0 ± 3311.8a
934.2 ± 59.0a
13944.3 ± 493.8a
5.9E+ 06 ± 4.6E+ 05a
1.3E+ 13 ± 1.5E+ 12
13727.2 ± 1595.5 410.8 ± 25.2a
58399.9 ± 3989.2a
900.1 ± 63.5a
13265.2 ± 350.6b
4.7E+ 06 ± 3.3E+ 05b
1.2E+ 13 ± 1.1E+ 12
440.7 ± 23.3b
70596.1 ± 5729.3b
1063.2 ± 79.8b
1.9 ± 0.7a+b 14231.8 ± 428.9a
5.8E+ 06 ± 6.3E+ 05a
1.5E+ 13 ± 1.9E+ 12a+b
450.4 ± 16.7 b+c
69834.2 ± 4804.4b
1098.8 ± 69.8b
1.9 ± 0.5a+b 14296.5 ± 726.0a
5.5E+ 06 ± 1.2E+ 06a
1.6E+ 13 ± 2.4E+ 12a+b 13498.0 ± 1846.6 483.6 ± 41.2 a+b
Table 2. Results for bone stiffness, bone strength and cortical bone micro-structural parameters
(Ct.Po, Ps.Pm, Ma.Ar, MMI, Ec.PM, Ct.Th) for each group evaluated. Means ± SD followed by: "a" represents
statistical y significant difference from ShOVX; "b" represents statistical y significant difference from the
OVX-shWBV group; and "c" represents statistical y significant difference between ALN-shWBV and ALN-
WBV (p < 0.05).
a compensatory mechanism after ovariectomy, a slight increase in Ps.Pm was observed in OVX-shWBV
compared to shOVX (+ 2.8%, Mechanical stimulation of OVX rats with HF WBV for 14 days
had no effect on the trabecular bone, except for TBPf (+ 23.5%, OVX-WBV versus OVX-shWBV
At cortical level, the mechanical treatment was able to partial y reverse the bone micro-structural deteri-
oration after ovariectomy by decreasing substantial y the Ma.Ar (-20.4%) and increasing Ct.Th (+ 7.2%)
(OVX-WBV versus OVX-shWBV). Additional y, it is noteworthy that there were no differences between
the cortical micro-structural parameters quantified for OVX-WBV and shO
Unlike the single HF loading treatment, the pharmacological treatment with alendronate revealed
a positive effect mainly on the trabecular bone micro-architecture of OVX rats, which was demon-
strated by an increase in BV (+ 107.8%), BV/TV (+ 120%) and Tb.N (+ 300%) and a decrease in Tb.Sp
(− 58.2%), TBPf (− 64.7%) and SMI (− 50.0%). However, ALN administration was not able to improve
the Tb.Th after ovariectomy (ALN-shWBV versus OVX-shWBVt cortical level, ALN adminis-
tration was associated with an increased Ct.Th (+ 9.8%) and Ct.Po (+ 60.8%) compared to OVX-shWBV
(ALN-shWBV versus OVX-shWBVhen compared to shOVX group, ALN also presented
higher values of Ct.Po (+ 38.8%), Ps.Pm (+ 5%), MMI (+ 18.5%) and Ma.Ar (+ 20.3%) (ALN-shWBV
versus shOal y, the combined application of ALN and HF resulted in a greater increase
in Ct.Th when compared to shOVX or ALN-shWBV groups (+ 7.1% for ALN-WBV versus shOVX and
+ 7.4% for ALN-WBV versus ALN-shWBV
Effect of WBV and/or ALN on bone mechanical competence. OVX caused a substantial decrease
in bone stiffness (− 10.7%) and bone strength (− 10.4%) compared to shOVX (OVX-shWBV versus
shOne mechanical treatment of OVX rats with HF loading via WBV (OVX-WBV)
did not affect bone stiffness nor strength when compared to OVX-shWBV. However, the pharmacologic
treatment with ALN, combined or not with WBV, significantly increased bone stiffness (+ 15.8% for
ALN-shWBV and 14.5% for ALN-WBV) and bone strength (+ 13.8% for ALN-shWBV and + 17.6%
for ALN-WBV) compared to the respective untreated OVX condition, with values equalling those of
the shOVX group. In addition, no significant differences were detected between ALN-shWBV and
ALN-WBV groups regarding bone stiffness and bone strengtn
Scientific RepoRts 5:10795 DOi: 10.1038/srep10795
The higher resolution of micro-computed tomography images and mechanical testing procedur
has highlighted the significance of several bone properties other than BMD for bone strengt, which
can improve the fracture risk prediction and the assessment of anti-fracture efficacy of osteoporosis
therapies. Impaired bone strength associated with altered bone turnover might result from decreases in
the amount of bone mass, changes in bone micro-architecture or geometry, in the biophysical properties
of bone tissue, or even from a combination of all of the abov. Therefore, the present study by using
three-dimensional analysis, μ CT and μ FE analyses, evaluated the potential relation of bone mechanical
competence with bone micro-structural changes of ovariectomy-induced compromised bone after single
or combined treatment with HF loading and bisphosphonates.
In OVX osteoporotic rats, a decreased bone mechanical competence associated with a cortex thin-
ning and trabecular bone loss was observed. This increased bone fragility is a result of the negative bone
multicelul ar unit balance induced by estrogen withdrawal after ovariectomy, which is the morphological
basis of bone loss and structural deterioratio. With a larger surface-to volume ratio, trabecular bone
is rapidly affected by increases in bone resorptionndividual trabeculae become progressively thinner,
shifting from a plate-like shape to a rod-like shape while trabecular separation increases. Progressive
perforation of individual rods leads to the loss of trabecular connectivity and reduces the number of
trabeculae, resulting in trabecular micro-architecture deteriorationese changes in trabecular
micro-architecture were also observed in previous studies with ovariectomized rats and rapidly compro-
mise bone strengtht cortical level, a higher endocortical resorption was noticed in OVX animals
represented by an increase in Ma.Ar compared to shOVX, leading to a reduction in bone mass and cor-
tical thickness despite of the slight periosteal expansion after ovariectomy. Clinical studies in postmeno-
pausal women also reported about 50% of cortical bone loss as a result of remodelling within the inner
(endosteal) surface adjacent to the marrow by cavitationeakening of the cortical bone compartment
is also of major importance for fragility fractures once cortical bone represents a substantial amount of
the total bone mass, especial y in the appendicular skeleto. These results therefore demonstrate that
micro-architectural changes after ovariectomy in either cortical or trabecular bone are responsible for
significant variations in the bone mechanical properties.
The mechanical treatment with HF loading was not able to oppose the negative effects of ovariec-
tomy on the bone mechanical competence. HF loading increased the cortical thickness of OVX rats by
decreasing the endocortical resorption, represented in this study by lower values of Ma.Ar compared to
OVX-shWBV. Notwithstanding this, the increase in cortical thickness after HF loading was not able to
improve the OVX bone strength and bone stiffness since this mechanical stimuli did not prevent the tra-
becular bone deterioration, as also demonstred by Hatori et a. In agreement with our results, a recent
study with ovariectomized rats also did not find substantial effects of mechanical stimulation neither on
bone biomechanical properties nor on trabecular bone micro-architecture (4 weeks of WBV loading)
However, after 12 weeks of mechanical treatment an improvement of the trabecular bone parameters of
OVX animals could be noticedese results are in contrast to those reported by Tezval et al.d
Sehmisch et al.ho found that treatment with WBV for 5 weeks resulted in improved bone strength
and bone mass, equalling the levels as observed in untreated sham rats. It is worth noting that differ-
ences could arise due to the skeletal site evaluated in the latter studies. The present study as well as the
study by Chen and co-workers investigated the effects of mechanical treatment at the tibia, while the
study of Tezval et a and Sehmisch et al.valuated the femur and vertebral body, respectively. The
tibia was chosen because this sites enables concomittantly trabecular and cortical bone analysis, which
is not the case for the vertebral body. Furthermore, the femura were used for isolation of bone-marrow
derived stromal cel s and investigation of the role of hormonal and mechanical influences on the pro-
genitor cel s' biology. Also, the response of the skeletal tissues to mechanical loading depends on several
factors including magnitude, duration and rate of stimuluence, it might be that the short duration
of experimental period used in the present study, namely 14 days, has been insufficient to increase the
bone mechanical competence in ovariectomized rats, since more densely mineralized bone is removed
after ovariectomy and replaced by younger less mineralized bone, which has reduced stiffness.
Unlike single HF loading treatment, alendronate administration was able to significantly enhance the
bone mechanical competence in ovariectomized rats. This might be attributed to the efficacy of this drug
in preventing ovariectomy-induced trabecular bone micro-architectural deteterioration. The large sur-
face area of trabecular bone is an advantage in therapeutics because it facilitates the access of biphospho-
nates to inhibit matrix remodelint the same time, a limited effect of ALN on the endocortical bone
remodeling was observed, since the drug was not able to decrease the Ma.Ar enlargement that occurs
after ovariectomy. As biphosphonates act by binding avidly to mineralized bone matrix, and consider-
ing that at intracortical or endocortical surfaces there is less volume of mineralized cortical bone matrix,
the accessibility of this drug to the bone remodeling that is occuring at these surfaces is reduced
Consequently, osteoclasts initiating remodeling upon a Haversian canal surface might not encounter and
engulf matrix containing drug and continue reabsorbing bone at endocortical surface, particularly in
rodents that lack a true Haversian canal syst. Nevertheless, the association of ALN with HF loading
seems to provide an additive effect at the level of endorcortical surface, demonstrated by lower values
of Ma.Ar in comparison to the single pharmacological treatment. Thus, the effect of HF stimuli on the
endocortical surface combined with the effect of ALN at the periosteal surface level contributed for an
Scientific RepoRts 5:10795 DOi: 10.1038/srep10795
enlarged cortical thickness when compared to single application of these treatments. Chen et al.lso
showed that WBV enhanced the effect of alendronate on the trabecular micro-architecture in ovariecto-
mized rats over 12 weeks, but the micro-structural changes at cortical level after this combined treatment
was not investigated. In the present study, although there is a trend of the combined treatment with
HF loading and ALN in improving trabecular micro-architecture and bone strength after ovariectomy,
the results were statistical y not significant when compared to single pharmacological treatment over 2
weeks. Therefore, long-term studies are needed in order to elicit the role of HF loading, combined or
not with ALN, on trabecular bone.
From a biomechanical point of view, an effective treatment for bone fragility should improve the
extrinsic biomechanical properties of bone but at the same time not substantial y impair the intrinsic
properties. However, it is rare for a treatment to achieve this combination of effec. Bisphosphonates,
by reducing excessively bone turnover, decreases the renewal of bone tissue and consequently increases
the accumulation of older and extensively mineralized bon. As a consequence, the bone becomes
more brittle and therefore unable to absorb energy by elastic deformation. Additional y, it leads to the
accumulation of damaged bone, facilitating micro-crack proliferation. Therefore, recent studies have
provided a well documented association between atypical fractures and long-term oral intake of alendro-
natehich is a growing clinical concerence, in order to achieve a safer and better clinical effect
on osteoporosis treatment, the combined use of ALN with HF WBV could be advised. Exercise–induced
improvements in the inorganic bone component does not seem to restrict the mineralization degree,
improves also the water conten, which is assumed to confer additional biomechanical advantag as
the tissue become less brittle and more able to accomodate the loads applied to the bone before devel-
oping micro-damage. This combination therapy might decrease the dosage of ALN in clinical patients.
Using a reduced dosage of biphosphonates (lower weekly dose or dosing every 2 weeks) is an unproven
but appealing alternativroviding an adequate but less complete surpress of bone turnovernd is
likely to provide fracture risk reduction.
The cross-sectional design and the relatively short-term experiment period of this study were the main
limitations. Therefore, it remains unclear whether longer-term changes on the bone micro-architecture
after HF and ALN interventions would still affect the bone mechanical competence. Furthermore, an
in silico technique was used in this study to determine the bone stiffness and bone strength based on
bone micro-architecture since this methodology was successfuly validated in previous studies showing
high precisionuture studies should also consider the inclusion of other methodologies to evaluate
the biophysical properties of bone tissue, such as the degree and type of col agen cross-linking, hydra-
tion, the mineral crystal size and their crystallinity in order to provide a complete insight into the bone
In conclusion, in comparison to single treatment with HF loading, ALN proved to be better in improv-
ing the mechanical competence of OVX-induced compromised bone. In addition, the association of ALN
with HF loading resulted in an additive anabolic effect on the cortical thickness.
1. Rachner, T. D., Khosla, S. & Hofbauer, L. C. Osteoporosis: now and the future. Lancet 377, 1276–1287 (2011).
2. Khosla, S., Oursler, M. J. & Monroe, D. G. Estrogen and the skeleton. Trends Endocrinol. Metab. 23, 576–581 (2012).
3. Pacifici, R. Estrogen, cytokines, and pathogenesis of postmenopausal osteoporosis. J. bone Miner. Res. 11, 1043–1051 (1996).
4. Johnel , O. & Kanis, J. A. An estimate of the worldwide prevalence and disability associated with osteoporotic fractures.
Osteoporos. Int. 17, 1726–1733 (2006).
5. Eastel , R., Walsh, J. S., Watts, N. B. & Siris, E. Bisphosphonates for postmenopausal osteoporosis. Bone 49, 82–88 (2011).
6. Lindsay, R., Watts, N. B., Lange, J. L., Delmas, P. D. & Silverman, S. L. Effectiveness of risedronate and alendronate on nonvertebral
fractures: an observational study through 2 years of therapy. Osteoporos. Int. 24, 2345–2352 (2013).
7. Siris, E. S. et al. Impact of osteoporosis treatment adherence on fracture rates in North America and Europe. Am. J. Med. 122,
S3–S13 (2009).
8. Aspenberg, P. & Schilcher, J. Atypical femoral fractures, bisphosphonates, and mechanical stress. Curr. Osteoporos. Rep. 12,
189–193 (2014).
9. Ettinger, B., Stuenkel, C. A. & Schnatz, P. F. Menopause practitioner perspective on the American Society of Bone and Mineral
Research Task Force report on atypical femoral fracture. Menopause 20, 1092–1097 (2013).
10. Ozcivici, E. et al. Mechanical signals as anabolic agents in bone. Nat. Rev. Rheumatol. 6, 50–59 (2010).
11. Judex, S. & Rubin, C. T. Is bone formation induced by high-frequency mechanical signals modulated by muscle activity?
J. Musculoskelet. neuronal Interact. 10, 3–11 (2010).
12. Judex, S., Lei, X., Han, D. & Rubin, C. Low-magnitude mechanical signals that stimulate bone formation in the ovariectomized
rat are dependent on the applied frequency but not on the strain magnitude. J. Biomech. 40, 1333–1339 (2007).
13. Delmas, P. D. & Seeman, E. Changes in bone mineral density explain little of the reduction in vertebral or nonvertebral fracture
risk with anti-resorptive therapy. Bone 34, 599–604 (2004).
14. Cummings, S. R. et al. Improvement in spine bone density and reduction in risk of vertebral fractures during treatment with
antiresorptive drugs. Am. J. Med. 112, 281–289 (2002).
15. Seeman, E. Is a change in bone mineral density a sensitive and specific surrogate of anti-fracture efficacy? Bone 41, 308–317
16. Dalle Carbonare, L. & Giannini, S. Bone microarchitecture as an important determinant of bone strength. J. Endocrinol. Invest.
27, 99–105 (2004).
17. Muller, R. & van Lenthe, G. H. Trabecular bone failure at the microstructural level. Curr. Osteoporos. Rep. 4, 80–86 (2006).
18. Mueller, T. L. et al. Computational finite element bone mechanics accurately predicts mechanical competence in the human
radius of an elderly population. Bone 48, 1232–1238 (2011).
19. Boyd, S. K., Szabo, E. & Ammann, P. Increased bone strength is associated with improved bone microarchitecture in intact female
rats treated with strontium ranelate: a finite element analysis study. Bone 48, 1109–1116 (2011).
Scientific RepoRts 5:10795 DOi: 10.1038/srep10795
20. Kilkenny, C., Browne, W. J., Cuthil , I. C., Emerson, M. & Altman, D. G. Improving bioscience research reporting: The ARRIVE
guidelines for reporting animal research. J. Pharmacol. Pharmacother. 1, 94–99 (2010).
21. Chatterjee, M. et al. High-frequency loading positively impacts titanium implant osseointegration in impaired bone. Osteoporos.
Int. 26, 281–290 (2015).
22. Bouxsein, M. L. et al. Guidelines for assessment of bone microstructure in rodents using micro-computed tomography. J. bone
Miner. Res. 25, 1468–1486 (2010).
23. Van Rietbergen, B., Weinans, H., Huiskes, R. & Polman, B. J. W. Computational Strategies for Iterative Solutions of Large Fem
Applications Employing Voxel Data. Int. J. Numer. Methods Eng. 39, 2743–2767 (1996).
24. Arbenz, P., van Lenthe, G. H., Mennel, U., Müller, R. & Sala, M. A scalable multi-level preconditioner for matrix-free μ -finite
element analysis of human bone structures. Int. J. Numer. Methods Eng. 73, 927–947 (2008).
25. Utkarsh, A. et al. ParaView 3.0 – A Paral el Visualization Application. (2007) Available accessed:
1st October 2014)
26. Pistoia, W. et al. Estimation of distal radius failure load with micro-finite element analysis models based on three-dimensional
peripheral quantitative computed tomography images. Bone 30, 842–848 (2002).
27. Wirth, A. J., Müller, R. & van Lenthe, G. H. Computational analyses of small endosseous implants in osteoporotic bone. Eur.
Cel s Mater. 20, 58–71 (2010).
28. Chappard, D., Baslé, M. F., Legrand, E. & Audran, M. New laboratory tools in the assessment of bone quality. Osteoporos. Int.
22, 2225–2240 (2011).
29. Donnel y, E. Methods for assessing bone quality: A review. in Clin. Orthop. Relat. Res. 469, 2128–2138 (2011).
30. Fonseca, H., Moreira-Gonçalves, D., Coriolano, H. J. A. & Duarte, J. A. Bone quality: The determinants of bone strength and
fragility. Sport. Med. 44, 37–53 (2014).
31. Seeman, E. & Delmas, P. D. Bone quality–the material and structural basis of bone strength and fragility. N. Engl. J. Med. 354,
2250–2261 (2006).
32. Seeman, E. Age-and menopause-related bone loss compromise cortical and trabecular microstructure. Journals Gerontol. 68,
1218–1225 (2013).
33. Boyd, S. K., Davison, P., Muller, R. & Gasser, J. A. Monitoring individual morphological changes over time in ovariectomized
rats by in vivo micro-computed tomography. Bone 39, 854–862 (2006).
34. Sehmisch, S. et al. Effects of low-magnitude, high-frequency mechanical stimulation in the rat osteopenia model. Osteoporos. Int.
20, 1999–2008 (2009).
35. Tezval, M. et al. Improvement of Femoral Bone Quality After Low-Magnitude, High-Frequency Mechanical Stimulation in the
Ovariectomized Rat as an Osteopenia Model. Calcif. Tissue Int. 88, 33–40 (2011).
36. Zebaze, R. M. et al. Intracortical remodelling and porosity in the distal radius and post-mortem femurs of women: a cross-
sectional study. Lancet 375, 1729–1736 (2010).
37. Hatori, K. et al. Single and combined effect of high-frequency loading and bisphosphonate treatment on the bone micro-
architecture of ovariectomized rats. Osteoporos. Int. 26, 303–310 (2015).
38. Chen, G. et al. Effect of Low-Magnitude Whole-Body Vibration Combined With Alendronate in Ovariectomized Rats: A
Random Controlled Osteoporosis Prevention Study. PLoS One 9, 1–8 (2014).
39. Wei, Q. S. et al. Effect of whole body vibration therapy on circulating serotonin levels in an ovariectomized rat model of
osteoporosis. Iran. J. Basic Med. Sci. 17, 62–68 (2014).
40. Seeman, E. The structural and biomechanical basis of the gain and loss of bone strength in women and men. Endocrinol. Metab.
Clin. North Am. 32, 25–38 (2003).
41. Roschger, P. et al. Alendronate increases degree and uniformity of mineralization in cancellous bone and decreases the porosity
in cortical bone of osteoporotic women. Bone 29, 185–191 (2001).
42. Roelofs, A. J. et al. Influence of bone affinity on the skeletal distribution of fluorescently labeled bisphosphonates in vivo. J. Bone
Miner. Res. 27, 835–847 (2012).
43. Turek, J. et al. Bisphosphonate binding affinity affects drug distribution in both intracortical and trabecular bone of rabbits.
Calcif. Tissue Int. 90, 202–210 (2012).
44. Lelovas, P. P., Xanthos, T. T., Thoma, S. E., Lyritis, G. P. & Dontas, I. A. The laboratory rat as an animal model for osteoporosis
research. Comp. Med. 58, 424–430 (2008).
45. Turner, C. H. Biomechanics of bone: Determinants of skeletal fragility and bone quality. Osteoporos. Int. 13, 97–104 (2002).
46. Boivin, G. & Meunier, P. J. Effects of bisphosphonates on matrix mineralization. J. Musculoskelet. Neuronal Interact. 2, 538–543
47. Mashiba, T. et al. Effects of suppressed bone turnover by bisphosphonates on microdamage accumulation and biomechanical
properties in clinical y relevant skeletal sites in beagles. Bone 28, 524–531 (2001).
48. Tan, S., Saseendar, S., Tan, B., Pawaskar, A. & Kumar, V. Ulnar fractures with bisphosphonate therapy: a systematic review of
published case reports. Osteoporos. Int. 26, 421–429 (2015).
49. Huang, T. H. et al. Endurance treadmill running training benefits the biomaterial quality of bone in growing male Wistar rats.
J. Bone Miner. Metab. 26, 350–357 (2008).
50. Nyman, J. S. et al. The influence of water removal on the strength and toughness of cortical bone. J. Biomech. 39, 931–938 (2006).
51. You, L., Sheng, Z.-Y., Chen, J.-Y., Pan, L. & Chen, L. The safety and efficacy of early-stage bi-weekly alendronate to improve bone
mineral density and bone turnover in chinese post-menopausal women at risk of osteoporosis. J. Int. Med. Res. 39, 302–310
The authors would like to thank the Fund for Scientific Research of Flanders (FWO Vlaanderen -
Postdoctoral researcher K. Vandamme), the Brazilian Science Without Borders Program (246131/2012-8
process, PhD student G. Camargos), and the Swiss National Supercomputing Centre (compute time) for
supporting this study.
K.V., J.D. and I.N. conceived and designed the research; G.C., K.V., P.B., H.V.L and A.D.B.C performed
the experiments and/or analyzed the data. G.C. wrote the manuscript. All authors have reviewed the
Competing financial interests: The authors declare no competing financial interests.
Scientific RepoRts 5:10795 DOi: 10.1038/srep10795
How to cite this article: Camargos, G. V. et al. Mechanical competence of ovariectomy-induced
compromised bone after single or combined treatment with high-frequency loading and
bisphosphonates. Sci. Rep. 5, 10795; doi: 10.1038/srep10795 (2015).
This work is licensed under a Creative Commons Attribution 4.0 International License. The
images or other third party material in this article are included in the article's Creative Com-
mons license, unless indicated otherwise in the credit line; if the material is not included under the
Creative Commons license, users will need to obtain permission from the license holder to reproduce
Scientific RepoRts 5:10795 DOi: 10.1038/srep10795
Source: http://www.reposip.unicamp.br/bitstream/REPOSIP/241958/1/wos_000355605100001.pdf
SDS: Doxycycline Hyclate Capsules, USP SAFETY DATA SHEET Identification Product Identifier: Doxycycline Hyclate Capsules, USP Synonyms: Doxycycline hydrochloride hemiethanolate hemihydrate National Drug Code (NDC): Recommended Use: Company: 1925 West Field Court, Suite 300 Lake Forest, Illinois 60045
Copyright 2007 by the American Psychological Association 2007, Vol. 7, No. 4, 745–754 Functional Magnetic Resonance Imaging Responses Relate to Differences in Real-World Social Experience Naomi I. Eisenberger, Shelly L. Gable, and Matthew D. Lieberman University of California, Los Angeles Although neuroimaging techniques have proven powerful in assessing neural responses, little is knownabout whether scanner-based neural activity relates to real-world psychological experience. A jointfunctional magnetic resonance imaging (fMRI)/experience-sampling study investigated whether individ-ual differences in neurocognitive reactivity to scanner-based social rejection related to: (a) moment-to-moment feelings of social rejection during real-world social interactions ("momentary social distress")and (b) the extent to which these momentary feelings corresponded with end-of-day global assessmentsof social disconnection ("end-of-day social disconnection"). Individuals who showed greater activity inregions associated with affective and pain processing (dorsal anterior cingulate cortex, amygdala,periaqueductal gray) during scanner-based social rejection reported feeling greater momentary socialdistress during their daily social interactions. In contrast, individuals who showed greater activity inregions associated with memory and self-referential memory encoding (hippocampus, medial prefrontalcortex) showed a stronger correspondence between momentary social distress and end-of-day socialdisconnection, such that greater momentary social distress was associated with greater end-of-day socialdisconnection. These findings complement previous work showing a dissociation between momentaryand retrospective reports of affect and suggest that these processes rely on dissociable neural systems.