Joomla.pa.ibf.cnr.it
Pharmacological upregulation of
h-channels reduces the excitability
of pyramidal neuron dendrites
Nicholas P. Poolos1–3, Michele Migliore4,5 and Daniel Johnston1
1 Division of Neuroscience and 2Department of Neurology, Baylor College of Medicine, One Baylor Plaza, Houston, Texas 77030, USA
3 Current address: Department of Neurology and Regional Epilepsy Center, University of Washington, 325 9th Avenue, Seattle, Washington 98104, USA4 Yale University School of Medicine, Section of Neurobiology, New Haven, Connecticut 06520, USA5 Permanent address: National Research Council, Institute of Advanced Diagnostic Methodologies, Palermo 90146, Italy
Correspondence should be addressed to D.J. ([email protected])
Published online: 15 July 2002, doi:10.1038/nn891
The dendrites of pyramidal neurons have markedly different electrical properties from those of the
soma, owing to the non-uniform distribution of voltage-gated ion channels in dendrites. It is thus
possible that drugs acting on ion channels might preferentially alter dendritic, but not somatic,
lishing Gr
excitability. Using dendritic and somatic whole-cell and cell-attached recordings in rat hippocampal
slices, we found that the anticonvulsant lamotrigine selectively reduced action potential firing from
dendritic depolarization, while minimally affecting firing at the soma. This regional and input-
specific effect resulted from an increase in the hyperpolarization-activated cation current (Ih), a
voltage-gated current present predominantly in dendrites. These results demonstrate that neuronal
excitability can be altered by drugs acting selectively on dendrites, and suggest an important role
for Ih in controlling dendritic excitability and epileptogenesis.
2002 Nature Pub
Many central nervous system (CNS) neurons have extensively
pocampal pyramidal neurons, markedly reducing AP firing
arborized dendrites on which they receive the majority of their
when initiated from dendritic depolarization, but minimally
synaptic contacts. Recent advances in electrophysiological tech-
affecting APs initiated from somatic depolarization. This effect
niques have shown that the apical dendrites of hippocampal
on dendritic excitability was not due to action on Na+ chan-
and neocortical pyramidal neurons have markedly different
nels, but rather to an increase in
Ih, a voltage-gated current
electrical properties from those of their corresponding soma-
present in high density in the dendrites. These results show
ta, and these differing properties are due to non-uniform dis-
that a drug can affect excitability and AP firing regionally with-
tributions and kinetics of voltage-gated channels. For example,
in a neuron, and provide evidence that
Ih is centrally involved
in hippocampal pyramidal neurons, the A-type transient K+
in modulating neuronal excitability and thus may influence
current (
IA) and the hyperpolarization-activated cation cur-
rent (
Ih; h-channel) are present in the dendrites at many-foldhigher densities than they are in the soma1–5. The non-
uniform distribution of voltage-gated channels affect signal
Actions of anticonvulsants on dendritic excitability
processing in the dendrites, altering the retrograde propaga-
Using somatic and dendritic recordings in hippocampal CA1
tion (or ‘back-propagation') of action potentials (APs), and
pyramidal neurons, we tested the hypothesis that anticonvul-
the integration of synaptic potentials.
sant drugs differentially affect the excitability of dendrites and
We reasoned that some drugs that affect ion channels might
somata. Bath application of LTG during prolonged current
act preferentially on dendritic voltage-gated channels, and thus
injection at the soma caused a modest reduction of repetitive
selectively alter excitability in one region of the neuron. One
AP firing (
Fig. 1a), consistent with previous findings9,10. When
such class of drugs consists of anticonvulsants known to act
a similar rate of AP firing was elicited from a dendritic injection
on Na+ channels, and includes phenytoin (PHT), carba-
site, however, LTG application markedly reduced or abolished
mazepine (CBZ) and lamotrigine (LTG). LTG is a structurally
AP firing (
Fig. 1b). Injection of a range of current steps that
novel anticonvulsant that is clinically effective on both partial-
produced 5–20 APs in 500 ms under control conditions
onset and primarily generalized seizures, as well as in psychi-
(
Fig. 1c) showed that LTG abolished AP firing for all but the
atric illnesses such as bipolar disorder6,7. Here we consider
highest amplitude current injections in that series. LTG had a
whether these drugs that act on the excitability of CNS neu-
disproportionate effect on AP firing elicited from dendritic
rons as studied with somatic recordings8 might also have some
depolarization as compared to that from the soma. The effect
unforeseen effects on dendritic excitability. We found that LTG
of LTG on dendritic AP firing was reversible with prolonged
had a selective effect on the excitability of the dendrites of hip-
(>30 min) return to control solution (data not shown).
nature neuroscience • volume 5 no 8 • august 2002
Fig. 1. Lamotrigine (LTG) selectively lowered dendritic excitability.
(
a) Action potential (AP) firing elicited by current injection at the soma
under control conditions (Ctrl) was modestly reduced by bath applica-
tion of LTG (50 µM). Resting potential, –68 mV. (
b) AP firing elicited
from dendritic current injection under control conditions was abolished
by LTG (50 µM). Resting potential, –64 mV. Dendritic recording dis-
tance, 190 µm. (
c) Dendritic current injections (150–250 pA for
500 ms), which under control conditions produced 5–20 APs, led to lit-
tle or no AP firing in the presence of LTG (100 µM). Resting potential,
–60 mV. Dendritic recording distance, 180 µm.
(PHT, 99 ± 4.5% of control,
n = 3,
P > 0.05; CBZ, 99 ± 6.2%,
n = 3,
P > 0.05).
In addition to affecting AP firing from the dendrites, bath
application of LTG caused a depolarization of resting potentialwhich was concentration-dependent. This effect was similar inthe soma and dendrites (50 µM LTG, 3.1 ± 0.26 mV,
n = 13; 100
µM LTG, 5.1 ± 0.78 mV,
n = 9). In quantifying the effect of LTGon AP firing, resting potential was compensated to the originalpre-drug level. When resting potential was not compensated,
the effect of LTG on AP firing from dendritic current injection
was somewhat reduced but still significantly different from con-
trol (
Fig. 2d, top traces; AP firing, 53 ± 6.5% of control,
n = 6,
P < 0.001). In contrast, LTG had no significant effect on AP fir-
lishing Gr
ing from somatic current injection when resting potential was
uncompensated (
Fig. 2d, bottom traces; 99 ± 13% of control,
n = 4,
P > 0.05). Thus, the steady-state depolarization induced by
We similarly tested the effects of PHT and CBZ on dendritic
LTG reduced the magnitude of the effect of LTG on AP firing by
excitability. Bath application of PHT (
Fig. 2a) or CBZ (
Fig. 2b)
moving resting potential closer to threshold. However, even
did not significantly affect AP firing elicited from dendritic
when this resting potential change was uncompensated, LTG
current injection. We quantified the effect of LTG, PHT and
continued to selectively affect AP firing elicited in the dendrites.
2002 Nature Pub
CBZ on AP firing by averaging the number of APs elicited by a
LTG has been shown to affect Na+ currents in a use-
series of four 500-ms current injections of progressively grad-
dependent manner9,10. We therefore explored whether the effect
ed amplitude producing 5–20 APs under control conditions,
of LTG on APs recorded in dendrites might be due to a reduc-
then repeating these same current injections under drug con-
tion of the amplitudes of back-propagating APs. Back-
ditions (
Fig. 2c). LTG was found to reduce AP firing from den-
propagating APs antidromically activated by 50-Hz trains of stim-
dritic current injection to 17 ± 5.7% of control values (
n = 8),
ulation under control conditions showed a progressive decline
compared to a much smaller reduction of AP firing elicited atthe soma (83 ± 9.3% of control,
n = 6;
P < 0.0001 compared
to value at the dendrites). In contrast, PHT and CBZ had nosignificant effect on AP firing from dendritic depolarization
Fig. 2. LTG, but not phenytoin or carbamazepine, lowered dendritic
excitability. (
a) AP firing from dendritic current injection was minimally
affected by bath application of phenytoin (PHT, 50 µM). Resting potential,
–62 mV. Dendritic recording distance, 200 µm. (
b) Carbamazepine (CBZ,
50 µM) similarly had a minimal effect on AP firing from dendritic current
injection. Resting potential, –67 mV. Dendritic recording distance,
210 µm. (
c) Group data showing the selective effect of LTG on dendritic
AP firing compared to its effect on somatic AP firing, and the minimal
effects of PHT and CBZ on dendritic AP firing. Drug concentrations and
number of observations for each condition: LTG dend, 50–100 µM,
n = 8;
LTG soma, 50–100 µM,
n = 5; PHT dend, 50–100 µM,
n = 3; CBZ dend,
25–50 µM,
n = 3. (
d) Dendritic AP firing shown in response to a 200-pA
current injection under control conditions (top traces) was reduced by
∼50% in the presence of LTG (100 µM) when resting potential was not
compensated to control levels. Resting potential, –61 mV (Ctrl) and
–58 mV (LTG). Dendritic recording distance, 180 µm. When the same
experiment was done using somatic current injection (bottom traces),
LTG (100 µM) had no significant effect on AP firing. Resting potential,
–66 mV (Ctrl) and –61 mV (LTG). (
e) Back-propagating APs in the den-
drites elicited by 50-Hz antidromic stimulation under control conditions
were not affected by addition of LTG (50 µM). Resting potential, –60 mV.
Dendritic recording distance, 220 µm.
nature neuroscience • volume 5 no 8 • august 2002
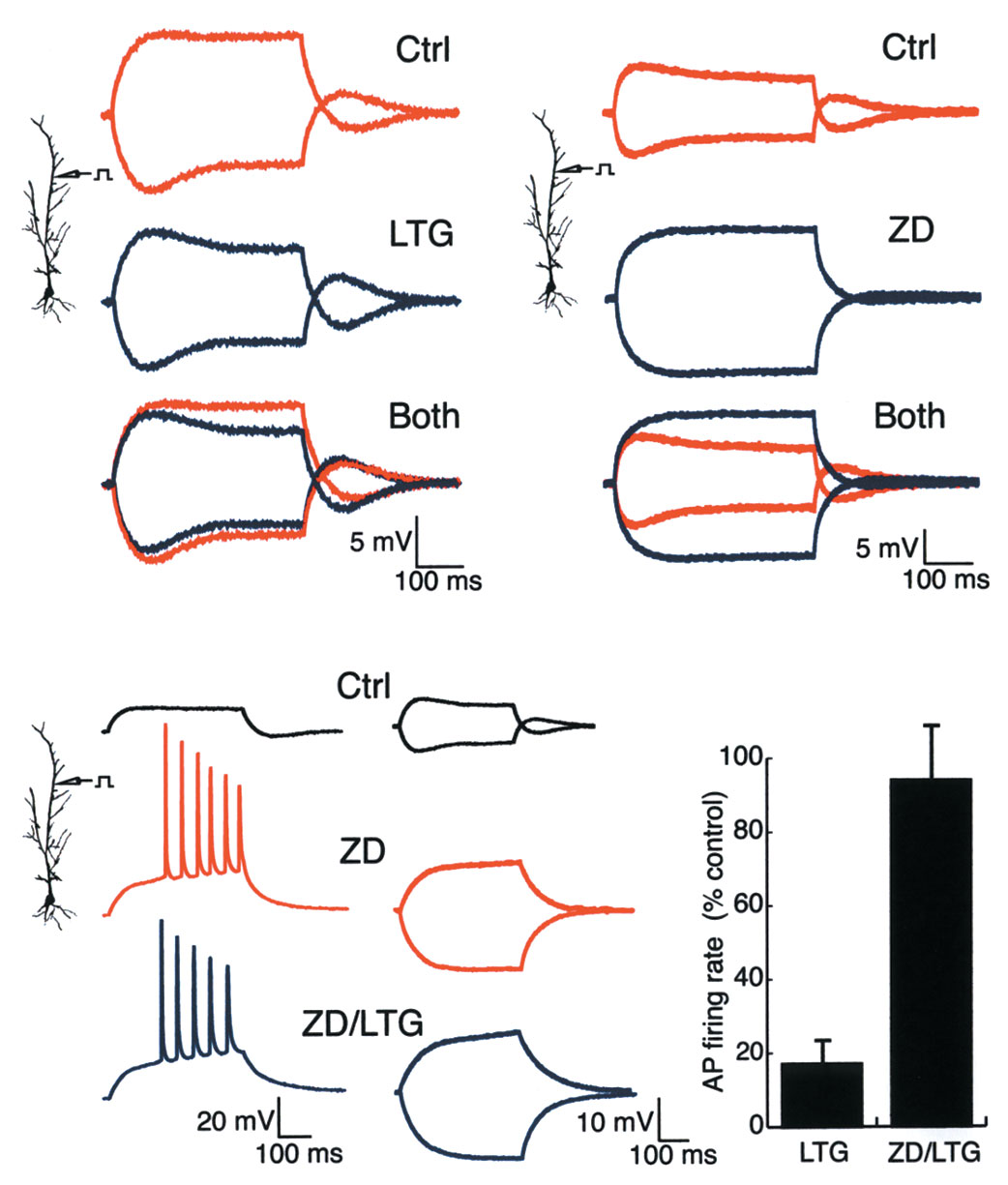
Fig. 3. LTG decreased AP firing elicited from the dendrites without
affecting AP back-propagation in dendrites. (a) Dual simultaneous
whole-cell recordings from soma and dendrites with dendritic current
injection. Under control conditions, APs elicited from dendritic current
injection initiated near the somatic recording site, then back-propagated
to the dendritic recording site, as shown by expanded traces with the
first APs from soma and dendrites (inset). In the presence of LTG
(100 µM), AP firing from dendritic current injection was reduced, but
APs continued to initiate near the somatic recording site and back-prop-
agate into the dendrites. Dendritic recording distance, 165 µm. Resting
potential for all traces, –64 mV. Calibration for insets is same as shown
for Fig. 3, but with voltage 40 mV and time 12.5 ms. (b) Same recording
as in (a), but with somatic current injection. LTG (100 µM) decreased AP
firing elicited from the soma to a much lesser extent than when elicited
from the dendrites, and AP back-propagation remained unaffected. APs
continued to initiate near the somatic recording site (inset).
in amplitude because of the slow inactivation of Na+ currents11
(Fig. 2e). LTG (50 µM) did not significantly affect the amplitudes
of antidromic APs throughout the train, including the first or
last APs in the train. The amplitude of the first back-propagat-ing AP in response to 50-Hz antidromic activation in the pres-ence of LTG (50–100 µM) was 97.3 ± 1.9 % (n = 6) of control APamplitude. Under control conditions, the amplitude of the tenth
lishing Gr
AP in the train decremented to 39.2 ± 3.4% (n = 6) of the ampli-tude of the first AP, while in the presence of LTG the tenth AP
not due to an effect on AP back-propagation per se, and was inde-
was 40.0 ± 4.5% (n = 6) of the first AP amplitude in LTG (not
pendent of actions on Na+ channels.
significantly different from control, P > 0.05). These data show
To explain how LTG could dramatically affect AP initiation
that LTG did not affect the amplitude of back-propagating APs
from dendritic current injection while minimally affecting APs
in the dendrites, nor their decrement due to dendritic Na+ cur-
elicited from the soma, we simultaneously made whole-cell
rent entering into a slow inactivated state. Because the ampli-
recordings from the soma and distal dendrites. When current
2002 Nature Pub
tudes of back-propagating APs in the dendrites are sensitive to
was injected into the dendrites under control conditions, APs
small changes in Na+ currents11, these results suggest that the
initiated near the somatic recording site (as determined by com-
action of LTG on reducing AP firing elicited in the dendrites was
paring the latency to onset of dendritic and somatic APs; Fig.
3a, inset), and then back-propagated into the dendrites (Fig. 3a,
Ctrl), consistent with prior studies12,13. In the presence of LTG,
Fig. 4. LTG decreased dendritic excitability by increasing activation of Ih.
(a) Whole-cell dendritic recordings showing subthreshold response to
both hyperpolarizing and depolarizing current injection. Under control
conditions (Ctrl), a slow relaxation of membrane potential (‘sag') in
response to both hyperpolarizing and depolarizing current injection
demonstrated the presence of Ih. In the presence of LTG (50 µM), the sag
was increased in response to both depolarizing and hyperpolarizing cur-
rent injection. Resting potential, –64 mV. Dendritic recording distance,
190 µm. (b) Application of ZD-7288 (ZD, 10 µM), a specific blocker of Ih,
blocked the sag in membrane potential and markedly increased input
resistance in response to current injection under control conditions, pro-
ducing an opposing effect on dendritic excitability compared to LTG.
Resting potential, –70 mV. Dendritic recording distance, 240 µm.
(c) Application of ZD-7288 (10 µM) caused an initially subthreshold den-
dritic current injection (left traces, Ctrl) to produce repetitive AP firing
(left traces, ZD). Subsequent application of LTG (50 µM) produced a min-
imal effect on dendritic AP firing in the presence of Ih blockade by ZD-
7288 (left traces, ZD/LTG). Similarly, the voltage sag produced by Ih with
subthreshold hyperpolarizing and depolarizing current injections under
control conditions (right traces, Ctrl) was blocked by ZD-7288 (right
traces, ZD). Adding LTG (50 µM) had no effect on the subthreshold
response in the presence of ZD (right traces, ZD/LTG). Resting potential,
–68 mV. Dendritic recording distance, 180 µm. (d) Group data showing
that the decrease in dendritic AP firing caused by LTG was largely blocked
by previous application of ZD-7288 (ZD/LTG). Drug concentrations and
number of observations for each condition: LTG, 50–100 µM, n = 8;
ZD/LTG, 50 µM LTG and 10 µM ZD-7288, n = 5.
nature neuroscience • volume 5 no 8 • august 2002
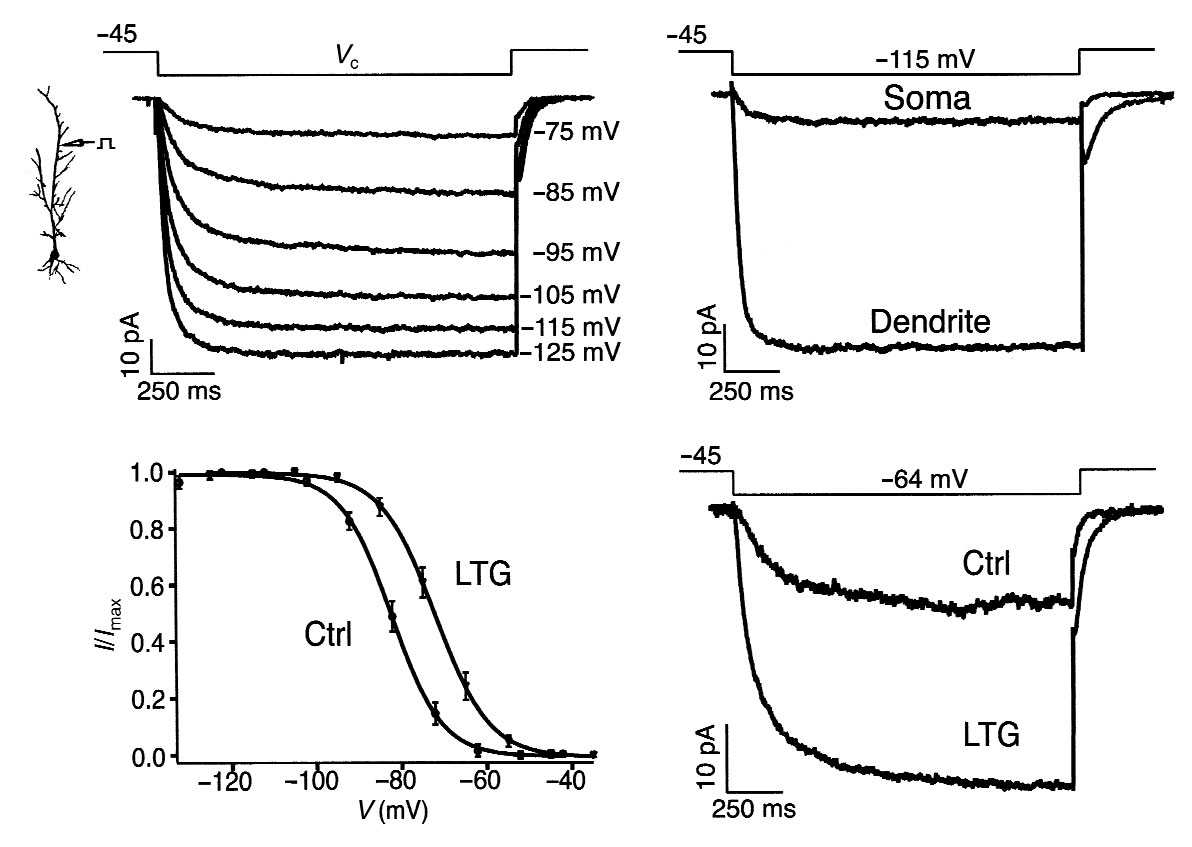
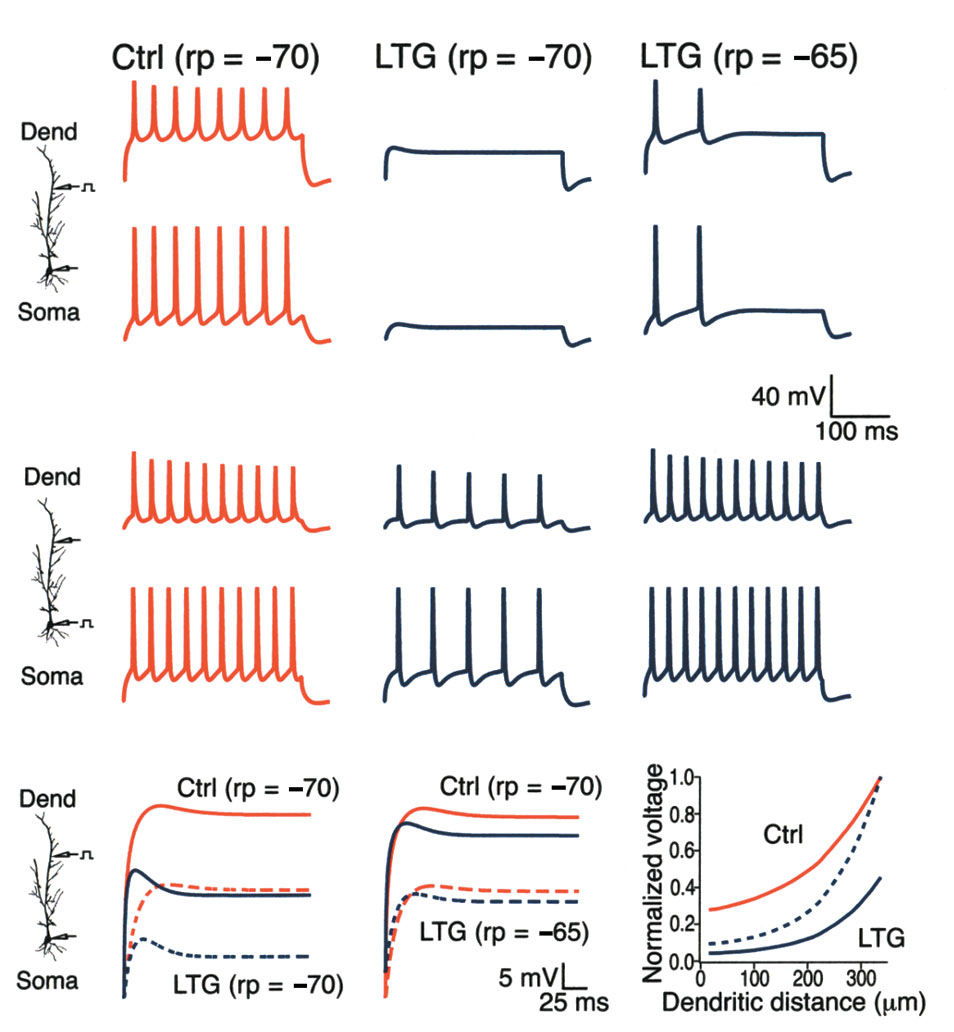
Fig. 5. LTG causes a depolarizing shift in Ih activation.
(a) Dendritic cell-attached patch recordings of Ih.
Hyperpolarizing voltage commands (Vc, labeled at right of
current traces) from a –45 mV holding potential produced
slowly activating inward currents characteristic of Ih.
Dendritic recording distance, 210 µm. (b) Ih recorded
from dendrites was markedly larger than that recorded at
the soma. Current traces shown are with a holding poten-
tial of –45 mV and a voltage command of –115 mV, and are
from different neurons; dendritic recording distance,
200 µm. (c) Ih activation from dendritic cell-attached
patch recordings. Addition of LTG (100 µM) to the pipette
solution produced an ∼11 mV depolarizing shift in Ih acti-vation (LTG) compared to control conditions (Ctrl; V1/2roughly –83 mV; V1/2 in LTG, roughly –72 mV). Number of
observations for each point in activation curves: control,
n = 7; LTG, n = 6. (d) Representative Ih from voltage com-
mands near rest in a neuron under control conditions
(Ctrl) and a separate neuron with LTG (100 µM) in the
recording pipette; to facilitate comparison, control cur-
rent trace was scaled such that maximal Ih was the same in
both control and LTG conditions.
AP firing was significantly reduced; however, APs still initiated
not altering the back-propagation of APs into the dendrites, but
near the somatic recording site and back-propagated into the
instead was decreasing the transmission of current injected from
lishing Gr
dendrites (Fig. 3a, LTG). When current was instead injected at
the dendrites.
the soma (Fig. 3b), APs continued to initiate near the soma (Fig.
3b, inset) but LTG had a much smaller effect on AP firing rate,
LTG affects subthreshold response of dendrites
and did not affect the back-propagation of APs into the den-
Prolonged hyperpolarizing and depolarizing subthreshold cur-
drites. (Note that the initial dendritic AP in the train was of sim-
rent pulses injected into the dendrites under control conditions
ilar amplitude in both control and drug conditions, but that the
produced voltage waveforms with a slowly activating relaxation
amplitude of subsequent APs varied with firing frequency,
(or ‘sag') that indicated the presence of the Ih current14,15. Ih is a
2002 Nature Pub
reflecting decrement due to frequency-dependent slow inacti-
slowly activating, non-inactivating inward current which is active
vation of Na+ channels11.) These results confirmed that LTG was
at resting potential and increases its activation with hyperpo-
Fig. 6. Ih activation decreased AP firing in a computational model. (a) Simulated AP firing in the dendrites (upper traces) and at the soma (lower
traces) in response to dendritic current injection. Responses are shown under control conditions with resting potential (rp) set at –70 mV, and dur-
ing increased Ih activation (10-mV shift) with rp set at –70 mV or –65 mV to simulate the depolarizing action of a 10-mV shift in Ih activation. A simu-
lated increase in Ih activation abolished AP firing from dendritic currentinjection when resting potential was held at control levels, and produced asignificant decrease in AP firing when resting potential was allowed to
depolarize to –65 mV. (b) Same conditions as in (a), but with somatic cur-
rent injection. A simulated increase in Ih activation produced a much
smaller effect on AP firing from somatic current injection than with den-
dritic current injection when resting potential was held at –70 mV. When
resting potential was held at –65 mV, increased Ih activation no longer
reduced AP firing from somatic current injection. Dendritic distance, 200
µm. (c) Simulated decay of voltage transients in response to a 700-pA step
current injection at 350 µm in the dendrites. When resting potential was
held at –70 mV, the steady-state voltage recorded in the dendrites under
control conditions (Ctrl; solid red line) was markedly reduced by a 10-mV
shift in Ih activation (LTG; solid blue line). The corresponding responses at
the soma (dashed lines) show an even larger proportional reduction in the
steady-state voltage. (d) Same responses as in (c), but with resting poten-
tial held at –65 mV to simulate the depolarization occurring with a 10-mV
shift in Ih activation. Under these conditions, the steady-state membrane
potential at the soma resulting from current injection in the dendrites was
still reduced compared to control. (e) Plot shows the steady-state depo-
larization from resting potential occurring along the dendrite produced by
a 650-pA current injection at 350 µm in the dendrites. Solid lines showdepolarization under either control (Ctrl) or increased Ih activation condi-tions (LTG; 10-mV shift) normalized to the peak depolarization at the siteof current injection under control conditions. Dashed line shows depolar-ization during increased Ih activation normalized to peak depolarization inthat condition. Resting potential, –70 mV in all traces.
nature neuroscience • volume 5 no 8 • august 2002
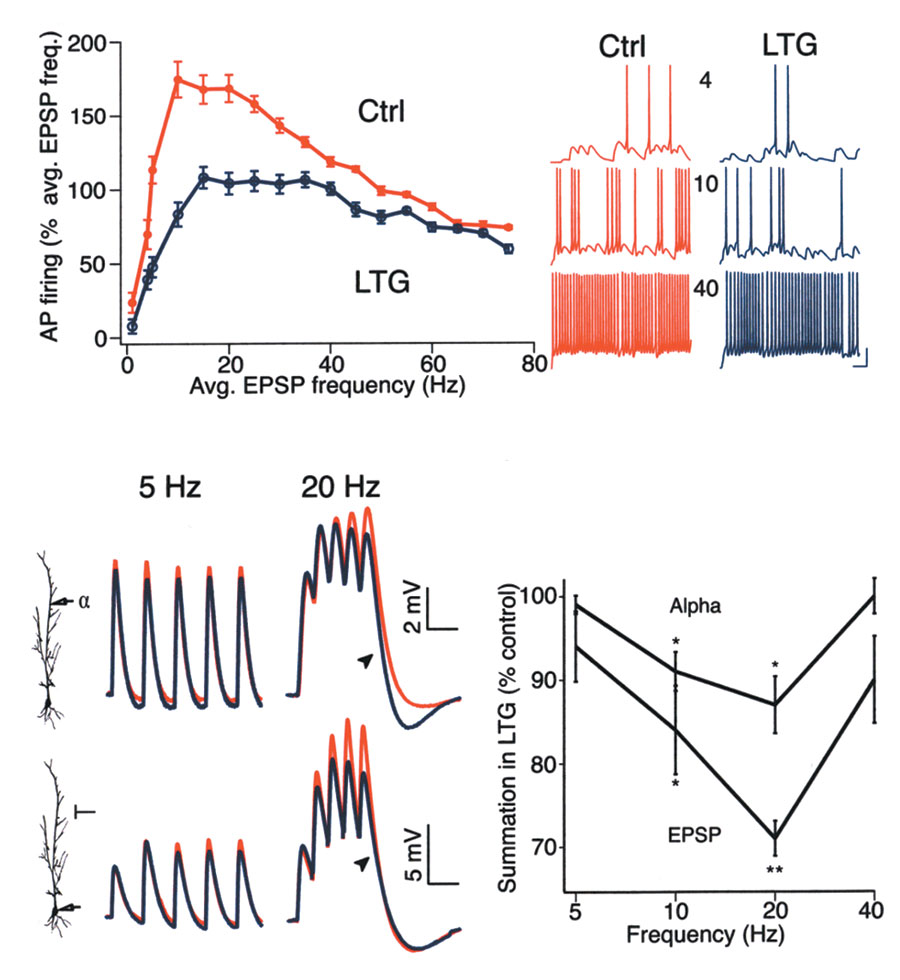
Fig. 7. LTG decreased synaptic activation of pyramidal neurons by
reducing temporal summation in a frequency-dependent manner.
(a) Simulated somatic AP firing in response to synaptic stimulation in the
dendrites. At low stimulus frequencies (<5 Hz), AP firing rate was mod-
estly affected by increased Ih activation (10-mV shift; LTG). As the stim-
ulus rate increased, AP firing rate under control conditions increased
rapidly due to temporal summation, but increased at a much slower rate
with increased Ih activation, with the greatest effect of increased Ih
occurring between 10 and 30 Hz. At higher frequencies (>30 Hz), the
effect of increased Ih diminished. Traces at right show sample AP firing in
control and increased Ih conditions at 4-, 10- and 40-Hz stimulation
rates. Resting potential, –65 mV for all traces. Calibration is 20 mV,
100 ms. (b) Experimental data showing superimposed dendritic
responses to current injection of alpha waveforms (upper traces) under
control (red traces) and LTG (100 µM; blue traces) conditions. LTG had
a minimal effect on a 5-Hz train of stimuli, but decreased temporal sum-mation for a 20-Hz train (arrowhead). Dendritic recording distance: 5 Hz, 240 µm; 20 Hz, 210 µm. Resting potential, –60 mV for both.
Somatic recordings of EPSPs resulting from stimulation of Schaffer col-laterals (lower traces) showed that LTG had a similar frequency-dependent effect on temporal summation as for alpha waveforms, mini-mally affecting low frequency EPSPs (5 Hz) but significantly reducing the
postsynaptic response to 20-Hz stimulation (arrowhead). Resting poten-
tial: 5 Hz, –61 mV; 20 Hz, –60 mV. Time calibration, 200 and 100 ms for
5 Hz and 20 Hz, respectively. (c) Plot of the reduction in temporal sum-
mation caused by LTG (100 µM) as measured with alpha waveform dendritic current injection or synaptic stimulation. LTG significantly
decreased temporal summation at 10 and 20 Hz, but not at 5 or 40 Hz. (Statistical significance compared to control, *P < 0.05 or **P < 0.005.)
lishing Gr
larization. Because of these unique properties, I
conditions became suprathreshold with repetitive AP firing in
h tends to stabi-
lize membrane potential towards rest, producing either a depo-
the presence of ZD-7288; when LTG was added, there was a min-
larizing sag in membrane potential with prolonged
imal effect on AP firing (Fig. 4c). Similarly, ZD-7288 abolished
hyperpolarizing current injection (reflecting activation of an
the effect of LTG on subthreshold current injection in the den-
2002 Nature Pub
inward current), or a hyperpolarizing sag in response to pro-
drites (Fig. 4c). Group data (Fig. 4d) shows that Ih blockade by
longed depolarizing current injection (due to deactivation of an
ZD-7288 prevented the effect of LTG on dendritic AP firing
inward current; Fig. 4a and b, Ctrl). This voltage sag thus
(94 ± 14% of control, n = 5, P > 0.05). These results suggest that
reduced steady-state input resistance in response to prolonged
LTG acted via Ih, increasing its steady-state activation at mem-
current injection. I
brane potentials near rest. This would have the effect of dimin-
h is distributed in the hippocampal pyramidal
neuron in a non-uniform gradient pattern, with a low density
ishing the dendritic input resistance, time constant and temporal
at the soma, and a density that increases up to sevenfold with
summation of excitatory inputs.
increasing distance in the apical dendrites16. The high dendrit-ic density of I
LTG increases activation of I
h contributes significantly to local integration prop-
erties, reducing the input resistance, time constant and temporal
We examined the mechanism of action of LTG on Ih using cell-
summation of EPSPs in the dendrites17,18.
attached patch recordings in the dendrites. This technique
In the presence of LTG, subthreshold current injection in the
allowed us to study Ih from a localized region of the dendrites,
dendrites produced responses with greater voltage sag, particu-
while leaving intact the modulation of this current by the intra-
larly in response to depolarization, suggestive of a larger I
cellular milieu20. Using a pipette solution that isolated I
rest (Fig. 4a). LTG thus reduced input resistance in the dendrites:
polarizing voltage commands from a holding potential of
when measured with 500-ms current injections producing
–45 mV produced slowly activating inward currents which were
<15 mV voltage excursions from rest, LTG (50–100 µM) low-
non-inactivating (Fig. 5a). The magnitude of these currents was
ered steady-state input resistance by 23.3 ± 5.2% compared to
small for voltage commands near rest (–75 mV) and progres-
control (n = 7, P < 0.005) in response to depolarizing current
sively increased with larger hyperpolarizing commands, as is typ-
injections, and by 13.6 ± 6.7% in response to hyperpolarizing
ical for Ih. The currents at the distal dendrites were far larger in
current injections (not statistically different from control,
peak amplitude than those at the soma (Fig. 5b). We plotted the
n = 5, P > 0.05). Consistent with the smaller density of I
voltage-dependent activation of I
h by measuring the peak inward
soma, however, LTG had a much smaller effect at the soma on
tail current upon return to holding potential after a 1-s voltage
the response to subthreshold current injection (data not shown).
command. Ih activation near resting potential (–60 to –70 mV)
Application of the specific I
was small under control conditions, and had a V
h blocker ZD-7288 at near-satu-
rating doses (10 µM)19 had the opposite effect (Fig. 4b). ZD-
–83 mV (Fig. 5c). Addition of LTG to the pipette solution pro-
7288 abolished the slowly activating voltage relaxation present
duced an 11-mV depolarizing shift in the Ih activation curve,
under control conditions in response to both hyperpolarizing
with a V1/2 of roughly –72 mV. This shift in Ih activation caused
and depolarizing current injection, and markedly increased input
a substantial increase in the amount of Ih active around resting
resistance. When ZD-7288 was pre-applied before LTG, it large-
potential (Fig. 5d). LTG did not increase maximal Ih measured
ly blocked the effect of LTG on dendritic excitability: sub-
at the most hyperpolarized potentials (Ih at –115 mV in control,
threshold current injections in the dendrites under control
30.0 ± 5.3 pA, n = 6; in LTG, 34.0 ± 6.3 pA, n = 8, P > 0.05). LTG
nature neuroscience • volume 5 no 8 • august 2002
also depolarized the resting potential, with an average depolar-
showed that voltage transients had an increased decay with dis-
ization of ∼5 mV upon break-in at the end of the experiment,
tance under conditions of increased Ih, demonstrating that
compared to control neurons. (This apparent shift in resting
increased Ih had lowered the length constant of the dendrite. This
potential is included in the 11-mV shift of Ih activation; that is,
suggested that the effects of LTG on somatic initiation of AP fir-
without accounting for the resting potential change, there was
ing from dendritic current injection were due to a decrease in
roughly a 6-mV activation shift.) We attribute this effect to prob-
input resistance at the site of current injection as well as to greater
able LTG entry into the dendritic membrane, affecting local Ih
attenuation of voltage transients along the length of the dendrite.
and thus local measurements of resting potential.
We then used the model to study the effects of LTG on synap-
tically driven AP firing, using a series of simulated random EPSP
LTG reduces synaptic activation of pyramidal neurons
trains delivered in the dendrites. Under control conditions, as
How does increased Ih activation affect AP firing in response to
stimulation frequency increased up to ∼20 Hz, the number of APs
repetitive synaptic stimulation, as would occur during epileptiform
elicited by EPSPs tended to increase, as temporal summation of
firing? To study how modulation of Ih would alter not only the post-
EPSPs increased (Fig. 7a). With a simulated increase in Ih activa-
synaptic response to trains of EPSPs in the dendrites but also the
tion, at low stimulation frequencies (<5 Hz) there was little change
transmission of voltage transients in the dendrites, we used a com-
in AP firing compared to control. However, at frequencies between
putational model (Methods) of the CA1 hippocampal pyramidal
10 and 30 Hz, higher Ih caused a significant reduction in synapti-
neuron. We first simulated AP firing from current injection in con-
cally evoked AP firing compared to control, presumably due to a
trol conditions and then with Ih activation shifted in a depolarized
decrease in temporal summation of EPSPs. At still higher fre-
direction by 10 mV. This simulated increase in Ih activation signif-
quencies, there was a decline in temporal summation under con-
icantly lowered the rate of AP firing from dendritic current injection,
trol conditions, and a similar decrease in the effect of increased
both when resting potential was held at its control level (Fig. 6a,
Ih. These results suggest that LTG might decrease the pyramidal
middle traces) and when resting potential was set 5 mV more depo-
neuron response to synaptic stimulation in a frequency-
larized, simulating the effects of LTG on resting potential (Fig. 6a,
dependent manner, with a peak effect between 10 and 30 Hz.
right traces). Conversely, simulated current injection at the soma
To investigate these computational phenomena experimen-
lishing Gr
showed only modest reduction of AP firing when Ih activation was
tally, we made dendritic whole-cell recordings and used short
increased and resting potential was fixed at control levels (Fig. 6b,
trains of alpha waveform current injections to simulate EPSPs.
middle traces); and when resting potential was held depolarized by
When trains of stimuli were delivered at low (5 Hz) frequency,
5 mV, increased Ih activation caused no reduction (or a small
there was little temporal summation, and application of LTG had
increase) in AP firing at the soma (Fig. 6b, right traces). This model
little effect on the extent of summation (Fig. 7b, top traces). At
replicated our experimental findings of a selective effect of increased
higher frequencies (10–20 Hz), there was more temporal sum-
Ih activation on dendritic excitability.
mation, and here LTG caused a decline in temporal summation
2002 Nature Pub
We then used the model to address questions more difficult to
during the train. This effect declined at higher frequencies (40 Hz;
study experimentally. Increased Ih activation seemed to have com-
Fig. 7c). When trains of synaptically evoked responses were sub-
peting influences on somatic AP firing in response to dendritic
stituted for alpha waveform current injections, and recordings
inputs: a reduction in dendritic input resistance reduced the depo-
made at the soma, LTG had little effect on low frequency stimu-
larization seen at the soma from an excitatory dendritic input,
lation (5 Hz), but markedly decreased temporal summation at
whereas the depolarizing change in resting potential brought the
higher frequencies (20 Hz; Fig. 7b, bottom traces). (Note that with
soma closer to threshold. We simulated subthreshold current injec-
short trains of EPSPs, temporal summation was superimposed
tions in the dendrites and found that, as expected, when resting
on synaptic facilitation, unlike the case with alpha waveform cur-
potential was held at the control level, increased Ih activation caused
rent injections.) Increased Ih caused a frequency-dependent
a significant decrease in the steady-state dendritic potential pro-
decrease in temporal summation which was of greatest magni-
duced by a dendritic current injection (Fig. 6c). However, the
tude around 20 Hz, and had little effect on low-frequency synap-
decrease in steady-state voltage seen at the soma was proportion-
tic activity (Fig. 7c). These results were in agreement with those
ately larger than in the dendrites, suggesting that the voltage tran-
obtained by computational modeling, and were similar whether
sient had more decay during its transmission from injection site
experimentally obtained with postsynaptic current injection or
to soma. When resting potential was set at a depolarized level dur-
synaptic stimulation, suggesting that the frequency-dependent
ing increased Ih activation, the resting potential change opposed
effects of increased Ih on synaptic activation of pyramidal neu-
some of the effects of Ih on dendritic input attenuation by mov-
rons were largely mediated postsynaptically.
ing closer to threshold. Even under these conditions, the steady-state membrane potential reached at the soma in response to a
dendritic input remained lower than control (Fig. 6d). This find-
These results demonstrate a new mode of action for CNS drugs.
ing showed that the effect of increased Ih on dendritic voltage atten-
LTG exerts an inhibitory effect on AP firing in the hippocampal
uation outweighed the effect of the resting potential change at the
pyramidal neuron by selectively altering the excitability of the api-
soma, and thus inhibited somatic AP firing from dendritic inputs.
cal dendrites while minimally affecting the excitability of the soma.
A simulation of the decay of voltage transients along the den-
This regional selectivity is a direct consequence of the drug's action
drite clarified how Ih preferentially attenuated dendritic inputs.
on Ih, which is distributed in a non-uniform gradient along the
We simulated a depolarizing current injection at a distal den-
neuron, with the distal dendrites containing a much higher den-
dritic location (350 µm), and measured the steady-state voltage
sity of Ih than the soma. Increases in dendritic Ih reduce input
along the length of the dendrite (Fig. 6e). A 10-mV shift in Ih
resistance, length constant and temporal summation, suppress-
activation caused steady-state voltage at the site of current injec-
ing the effect of dendritic excitatory synaptic inputs on periso-
tion to be reduced to ∼45% of control, but at the soma, the volt-
matic AP initiation in the pyramidal neuron, thus causing an
age transient had decayed to ∼15% of the control value. A plot
overall reduction in excitability. These regional effects of LTG
of the normalized steady-state voltage (Fig. 6e, dashed line)
within a single neuron demonstrate that drugs may have unex-
nature neuroscience • volume 5 no 8 • august 2002
pected and differing effects on different compartments of the neu-
of its efficacy against primarily generalized epilepsy (such as
ron, in particular on the dendritic tree (which by some estimates
absence epilepsy), a feature not shared by other anticonvulsants
accounts for >90% of the surface area of pyramidal neurons21),
with actions on Na+ channels such as PHT or CBZ24. A further
underscoring the importance of studying dendritic physiology
implication of these results is that Ih may have an important role
when evaluating pharmaceutical mechanisms of action.
in epileptogenesis, as is supported by recent evidence demon-
LTG seems to act by shifting the activation of Ih in a depolar-
strating alterations in Ih in an animal model of febrile seizures25,
izing direction. Because there is little Ih active at the typical neu-
and the involvement of Ih in maintaining the spontaneous activ-
ronal resting potential, small increases in Ih can lead to significant
ity of CA1 hippocampal interneurons26 and modulating presy-
modulation of subthreshold neuronal behavior. The contribu-
naptic excitability27. These multiple actions of Ih, some of which
tions of Ih to the integrative properties of dendrites include a
may increase rather than decrease neuronal excitability, imply that
reduction in input resistance, dendritic length constant, and tem-
its effects on epileptogenesis may be complex. Nonetheless, our
poral summation, all of which act to reduce dendritic excitabili-
results, along with the growing appreciation for the important
ty16–18. The reduction of dendritic input resistance and length
role of Ih in regulating neuronal excitability, suggest that Ih may
constant causes a given excitatory input in the dendrites to pro-
be a useful target for further CNS drug development.
duce less of a local depolarization which then decays with distancealong the dendrites. This produces a smaller voltage change at the
soma, ultimately reducing AP firing in response to dendritic depo-
Electrophysiology. Hippocampal slices (400 µm) were prepared from
larization. Increases in I
6–10 week-old male Sprague-Dawley rats using standard procedures4.
h also reduce temporal summation, dimin-
ishing AP firing from repetitive synaptic inputs in the dendrites.
Animal protocols were approved by the Animal Research Committee at
This probably results from the gradual buildup of I
Baylor College of Medicine. Neurons were visualized with differential
interference contrast microscopy using a Zeiss Axioskop (Oberkochen,
during a train of EPSPs, producing a net hyperpolarizing cur-
Germany). Recordings were made at 31–33°C. The extracellular recording
rent18. The frequency-dependence of this effect may derive from
solution contained 125 mM NaCl, 25 mM NaHCO
the relatively slow activation and deactivation kinetics of I
3, 10 mM dextrose,
2.5 mM KCl, 2 mM CaCl2, 2 mM MgCl2 and 1.25 mM NaH2PO4. CNQX
ms near rest16). Thus, low-frequency EPSPs do not achieve sig-
(10 µM) and bicuculline methiodide (20 µM) were added to the extra-
lishing Gr
nificant steady-state Ih deactivation, whereas higher frequencies
cellular solution except when synaptic stimulation was used. The whole-
(∼20 Hz) will lead to increased Ih deactivation and therefore
cell recording pipette solution contained 120 mM potassium gluconate,
decreased temporal summation. (At still higher frequencies the
20 mM KCl, 10 mM HEPES, 4 mM Na2-ATP, 2 mM MgCl2, 0.3 mM Tris-
GTP and 0.2 mM EGTA (pH 7.3 with KOH). For cell-attached patch
h effect may be overcome by increasing EPSP summation.)
Increases in I
recordings, the pipette solution contained 120 mM KCl, 20 mM TEA-Cl,
h also depolarize resting potential, moving it
towards threshold for AP firing, thus counteracting some of the
10 mM HEPES, 5 mM 4-aminopyridine, 2 mM CaCl2, 1 mM MgCl2 and1 mM BaCl
inhibitory effect on excitatory inputs. Our experiments and sim-
2 (pH 7.3 with KOH). Recorded neurons had resting poten-
tials between –60 and –72 mV (mean –64
2002 Nature Pub
± 0.7 mV, n = 36).
ulations showed that the balance of these two influences is differ-
Whole-cell current-clamp recordings were made using a Dagan BVC-
ent in the two compartments of the neuron. In response to
700 amplifier (Minneapolis, Minnesota), were sampled at 10 KHz, and fil-
somatic inputs, when the change in resting potential was experi-
tered at 2 KHz. Patch recordings used an Axon Instruments AxoPatch
mentally uncompensated (as would occur in vivo), LTG had lit-
1C amplifier (Foster City, California), and were sampled at 2 KHz and
tle or no effect on AP firing, suggesting that the small change in
filtered at 500 Hz. Data acquisition used custom software written for the
local input resistance was counterbalanced by the depolarization
Igor Pro analysis environment (Wavemetrics, Lake Oswego, Oregon).
of resting potential (which was more uniform throughout the
Extracellular stimulation in the alveus with a tungsten electrode (A-M
neuron). Increased I
Systems, Everett, Washington) was used to antidromically activate APs,
h had a more dramatic influence on dendrit-
and stimulation in the stratum radiatum was used to elicit EPSPs. Den-
ic inputs and their ability to drive somatic firing, and this
dritic current injections simulating EPSPs were modeled by an alpha
remained inhibitory even when the resting potential change was
function of the form: I = I
uncompensated. This is because increased I
max(t/α)e–αt where α = 0.1.
h not only had a much
Drugs were applied in the bath made either from aqueous stock solu-
larger effect on the local depolarization caused by a dendritic input
tions (LTG, ZD-7288) or dissolved in DMSO such that the final DMSO
(owing to its increased dendritic density), but also further atten-
concentration was <1% (PHT, CBZ). All drugs were obtained from Sigma
uated that potential during its spread from the dendrites to the
(St. Louis, Missouri) except as noted. LTG was a gift from GlaxoSmithKline
soma. These two effects combined to substantially reduce the
(Research Triangle Park, North Carolina); ZD-7288 was obtained from
effects of dendritic inputs on somatic AP firing, while minimally
Tocris (Balwin, Missouri). Concentration ranges of anticonvulsants used in
affecting somatic inputs. Thus it appears that increased activation
this study were chosen with reference to the established peak therapeuticfree cerebrospinal fluid concentrations in humans28: 39 µM LTG, 7 µM
of Ih by LTG reduces the overall excitability of hippocampal pyra-
PHT and 13 µM CBZ. Synaptic stimulation experiments were performed
midal neurons by attenuating their response to dendritic inputs.
with addition to the bath of 50 µM APV, 20 µM MK-801, 100 µM CGP-
The action of LTG on Ih may constitute an important new anti-
35348 (Tocris) and 20 µM bicuculline methiodide (to block NMDA and
convulsant mechanism. The frequency-dependent effect of LTG
GABAergic currents). Area CA3 was isolated from the rest of the slice by a
on temporal summation of EPSPs allows for the selective reduc-
knife cut to diminish repetitive firing from reduced GABAergic inhibition.
tion of AP firing that results from pathologically high levels of
In addition, EGTA (10 mM) was added to the pipette solution to reduce
excitatory synaptic activity in the dendrites, while preserving AP
potentiation of postsynaptic responses from repetitive synaptic stimula-
firing from lower frequency inputs. This fulfills a criterion for
tion. Temporal summation was measured as the ratio of fifth responseamplitude (measured from baseline) to the first response amplitude.
ideal anticonvulsant action: that normal brain function be unaf-
Group data are expressed as mean ± s.e.m. Statistical significance was
fected while excessive firing is suppressed. Other mechanisms of
calculated using Student's t-test.
action have been ascribed to LTG that may contribute to its anti-convulsant effect, including reduction of Na+ currents, Ca2+ cur-
Modeling. All simulations were carried out using the NEURON simula-
rents and glutamate receptor activation9,10,22,23. However, the
tion program29. The realistic model of a hippocampal CA1 pyramidal
action of LTG on I
neuron was based on that used in a previous work30 and included Na+,
h, and the role of Ih in modulating oscillatory
behavior in other neurons15 may provide a unique explanation
DR- and A-type K+ voltage-gated conductances. A non-inactivating, non-
nature neuroscience • volume 5 no 8 • august 2002
specific cation current Ih = gh*n*(V – Erev), with Erev = –30 mV was
8. White, H. S. in The Epilepsies 2 (eds. Porter, R. J. & Chadwick, D.) 1–30
inserted in the soma and the apical dendrites. Its dendritic distribution
(Butterworth-Heinemann, Boston, 1997).
and activation kinetics were consistent with the available experimental
9. Kuo, C.-C. & Lu, L. Characterization of lamotrigine inhibition of Na+
data on CA1 neurons16. Thus, the voltage dependence of the activation
channels in rat hippocampal neurons. Br. J. Pharmacol. 121, 1231–1238
(1997).
gate variable was modeled as n = 1/(1 + exp(0.151(V – V1/2))), with V1/2
10. Xie, X., Lancaster, B., Peakman, T. & Garthwaite, J. Interaction of the
= –82 mV in the soma and proximal dendrites (<100 µm), and V1/2 =
antiepileptic drug lamotrigine with recombinant rat brain type IIA Na+
–90 mV for locations >100 µm from the soma; its time constant was
channels and with native Na+ channels in rat hippocampal neurons.
approximated as α
Pflugers Arch. 430, 437–446 (1995).
n = exp(0.033(V – Vt))/(0.011(1 + exp(0.083(V – Vt)))),
11. Colbert, C. M., Magee, J. C., Hoffman, D. A. & Johnston, D. Slow recovery
t = –75 mV. A peak conductance density of gh = 3 pS/µm2 was
used at the soma, and linearly increased with distance, d (
µm), as g
from inactivation of Na+ channels underlies the activity-dependent
attenuation of dendritic action potentials in hippocampal CA1 pyramidal
1.5d/100). LTG application was modeled with a 10-mV depolarizing shift
neurons. J. Neurosci. 17, 6512–6521 (1997).
of the activation curve. The resting potential change resulting from this
12. Golding, N. L. & Spruston, N. Dendritic sodium spikes are variable triggers
shift in I
of axonal action potentials in hippocampal CA1 pyramidal neurons.
h activation was modeled as a fixed 5-mV depolarization. Synap-
tic conductances were represented by a double exponential function with
Neuron 21, 1189–1200 (1998).
rise and decay time constants of 3 and 30 ms, respectively, a peak con-
13. Stuart, G., Spruston, N., Sakmann, B. & Hausser, M. Action potential
initiation and backpropagation in neurons of the mammalian CNS. Trends
ductance of 16 nS, and a reversal potential of 0 mV. For the simulations
Neurosci. 20, 125–131 (1997).
of Fig. 7a, five independent synapses were randomly placed and ran-
14. Santoro, B.
Molecular and functional heterogeneity of
domly (poisson) activated on dendritic compartments 150–250 µm from
hyperpolarization-activated pacemaker channels in the mouse CNS.
the soma. The average AP firing rate at each average frequency was cal-
J. Neurosci. 20, 5264–5275 (2000).
culated from 50 simulations, each 1 s long. The model and simulation
15. Pape, H.-C. Queer current and pacemaker: the hyperpolarization-activated
cation current in neurons. Annu. Rev. Physiol. 58, 299–327 (1996).
files are publicly available on the ModelDB database of Senselab
16. Magee, J. C. Dendritic hyperpolarization-activated currents modify the
integrative properties of hippocampal CA1 pyramidal neurons. J. Neurosci.
18, 7613–7624 (1998).
17. Stuart, G. & Spruston, N. Determinants of voltage attenuation in
neocortical pyramidal neuron dendrites. J. Neurosci. 18, 3501–3510 (1998).
We thank R. Gray for writing the data acquisition software and providing help
18. Magee, J. C. Dendritic Ih normalizes temporal summation in hippocampal
CA1 neurons. Nat. Neurosci. 2, 508–514 (1999).
in all phases of experimentation and data analysis. We also thank R. Chitwood,
19. Harris, N. C. & Constanti, A. Mechanism of block by ZD 7288 of the
lishing Gr
C. Bernard and A. Frick for reading earlier versions of the manuscript. Research
hyperpolarization-activated inward rectifying current in guinea pig
was supported by the National Institutes of Health (N.P.P. and D.J.), the
substantia nigra neurons in vitro. J. Neurophysiol. 74, 2366–2378 (1995).
20. Chen, S., Wang, J. & Siegelbaum, S. A. Properties of hyperpolarization-
National Institute of Deafness and Other Communication Disorders Human
activated pacemaker current defined by coassembly of HCN1 and HCN2
Brain Project (M.M.), the National Epifellows Foundation (N.P.P.) and the
subunits and basal modulation by cyclic nucleotide. J. Gen. Physiol. 117,
Hankamer Foundation (D.J.).
491–504 (2001).
21. Fiala, J. C. & Harris, K. M. in Dendrites (eds. Stuart, G., Spruston, N. &
Hausser, M.) (Oxford University Press, Oxford, 1999).
Competing interests statement
22. Stefani, A., Spadoni, F., Siniscalchi, A. & Bernardi, G. Lamotrigine inhibits
2002 Nature Pub
Authors declare competing financial interests: see the Nature Neuroscience
Ca2+ currents in cortical neurons: functional implications. Eur. J.
Pharmacol. 307, 113–116 (1996).
website (http://neuroscience.nature.com) version for details.
23. Wang, S. J., Sihra, T. S. & Gean, P. W. Lamotrigine inhibition of glutamate
release from isolated cerebrocortical nerve terminals (synaptosomes) by
RECEIVED 12 FEBRUARY; ACCEPTED 25 JUNE 2002
suppression of voltage-activated calcium channel activity. Neuroreport 12,
2255–2258 (2001).
24. Coulter, D. A. Antiepileptic drug cellular mechanisms of action: where does
1. Hausser, M., Spruston, N. & Stuart, G. J. Diversity and dynamics of
lamotrigine fit in? J. Child Neurol. 12 (Suppl.1), 2–9 (1997).
dendritic signaling. Science 290, 739–744 (2000).
25. Chen, K. et al. Persistently modified h-channels after complex febrile
2. Johnston, D., Magee, J. C., Colbert, C. M. & Christie, B. R. Active properties
seizures convert the seizure-induced enhancement of inhibition to
of neuronal dendrites. Annu. Rev. Neurosci. 19, 165–186 (1996).
hyperexcitability. Nat. Med. 7, 331–337 (2001).
3. Magee, J., Hoffman, D., Colbert, C. & Johnston, D. Electrical and calcium
26. Lupica, C. R., Bell, J. A., Hoffman, A. F. & Watson, P. L. Contribution of the
signaling in dendrites of hippocampal pyramidal neurons. Annu. Rev.
hyperpolarization-activated current (Ih) to membrane potential and GABA
Physiol. 60, 327–346 (1998).
release in hippocampal interneurons. J. Neurophysiol. 86, 261–268 (2001).
4. Poolos, N. P. & Johnston, D. Calcium-activated potassium conductances
27. Beaumont, V. & Zucker, R. S. Enhancement of synaptic transmission by
contribute to action potential repolarization at the soma but not the
cyclic AMP modulation of presynaptic Ih channels. Nat. Neurosci. 3,
dendrites of hippocampal CA1 pyramidal neurons. J. Neurosci. 19,
133–141 (2000).
5205–5212 (1999).
28. Shorvon, S. D. Handbook of Epilepsy Treatment (Blackwell, Oxford, 2000).
5. Johnston, D. et al. Dendritic potassium channels in hippocampal pyramidal
29. Hines, M. L. & Carnevale, N. T. The NEURON simulation environment.
neurons. J. Physiol. (Lond.) 525, 75–81 (2000).
Neural Comput. 9, 1179–1209 (1997).
6. Messenheimer, J. in The Treatment of Epilepsy: Principles and Practice (ed.
30. Migliore, M., Hoffman, D. A., Magee, J. C. & Johnston, D. Role of an A-type
Wyllie, E.) 899–905 (Williams and Wilkins, Baltimore, 1996).
K+ conductance in the back-propagation of action potentials in the
7. Calabrese, J. R. Lamotrigine and the treatment of bipolar disorder.
dendrites of hippocampal pyramidal neurons. J. Comput. Neurosci. 7, 5–15
Introduction. Eur Neuropsychopharmacol. 9 (Suppl. 4), S107–108 (1999).
nature neuroscience • volume 5 no 8 • august 2002
Source: http://joomla.pa.ibf.cnr.it/personale/migliore/PDF/lamotrigine.pdf
REPUBLIC OF RWANDA MINISTRY OF DISASTER MANAGEMENT AND REFUGEE AFFAIRS NATIONAL CONTINGENCY PLAN FOR EARTHQUAKE Kigali, 2014 PREAMBLE A well-coordination of disaster and emergency response is a key factor of success in terms of life saving and property protection. One of the coordination tools is a plan, not only a document but and especially the planning processes which improve the possibility to reduce the uncertainty that may be fatal in responding to a disaster or an emergency
return to itm online CHECKING FOR POSSIBLE HERB-DRUG by Subhuti Dharmananda, Ph.D., Director, Institute for Traditional Medicine, Portland, Oregon The issue of herb-drug interactions looms large over the practice of herbal medicine. Up to now there have been very few incidents recorded of herb-drug interactions, but since the first such reports emerged a decade ago,a concern has been raised: that we know so little about herbs and their potential for interaction with drugs thatthese incidents could be just the "tip of the iceberg." Virtually all medical writers who review the literatureacknowledge the small number of reports, but conclude that the issue of herb-drug interactions is a serious onethat must be pursued. In a few instances, the interactions may have been responsible for severe consequences.